Engineers know the limitations of three dimensions
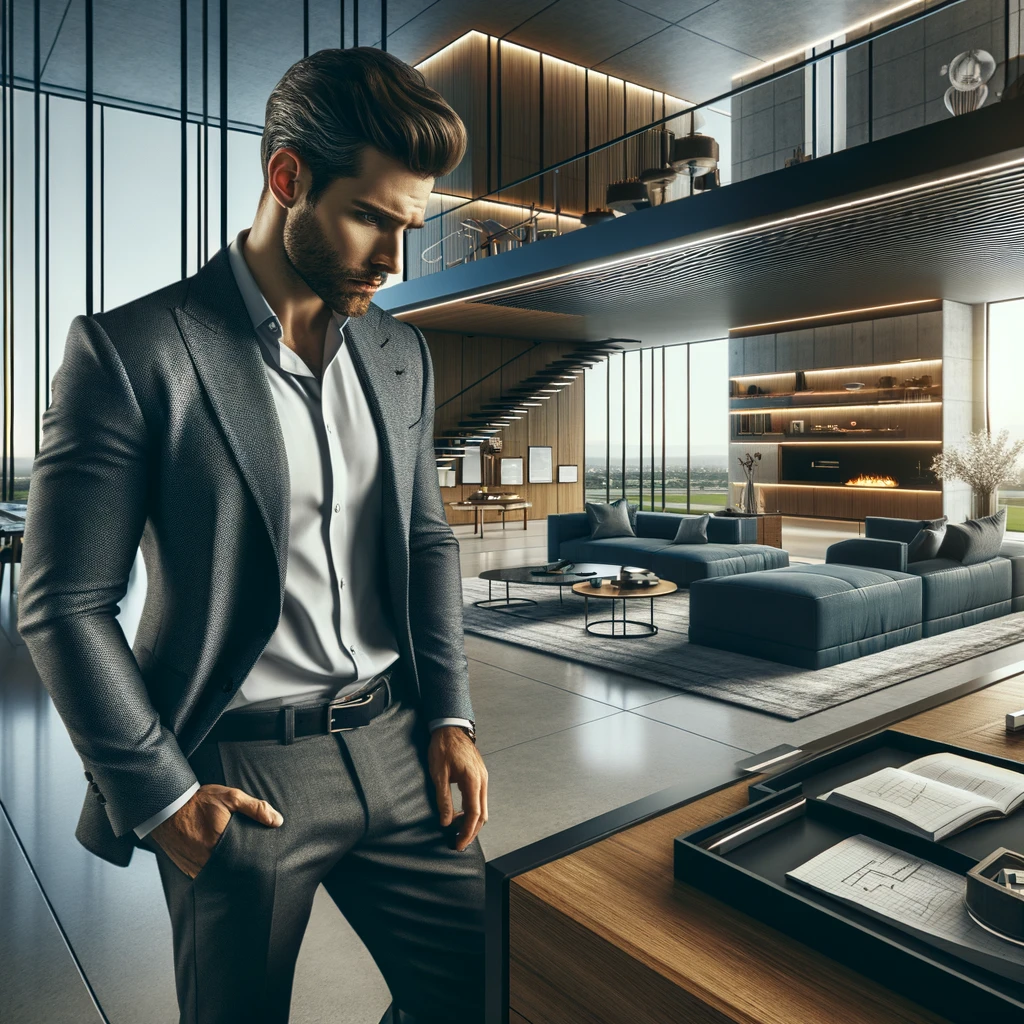
When we talk about the first three dimensions, we’re typically referring to the spatial dimensions of length, width, and height. While these dimensions provide a framework for understanding the physical extent of objects in space, they offer only a limited perspective on the full reality of what we observe. Here’s why:
Mass, Weight, and Density: The first three dimensions don’t inherently convey information about the mass, weight, or density of an object. These properties are related to the amount of matter contained within an object, which can vary independently of its size or spatial dimensions.
Speed and Trajectory: Understanding the speed and trajectory of an object involves factors beyond its spatial dimensions. It requires knowledge of its motion through space over time, which involves considering additional dimensions related to velocity, acceleration, and time itself.
Energy and Stability: Energy is a concept that goes beyond spatial dimensions and encompasses various forms such as kinetic energy, potential energy, and thermal energy. Stability, similarly, depends on factors such as the distribution of mass, the arrangement of atoms or molecules, and the forces acting on the object.
Gravity and Strength: Gravity is a fundamental force that affects objects regardless of their spatial dimensions. It depends on the masses of the objects involved and the distance between them, rather than their size or shape. Strength, on the other hand, relates to the internal structure and composition of materials, which may not be evident from their external dimensions alone.
Endurance and Other Qualities: Qualities like endurance, flexibility, or resilience involve aspects of behavior, performance, or response to external stimuli, which go beyond the static representation provided by the first three dimensions. They often require consideration of dynamic processes, interactions, and responses over time.
In essence, while the first three spatial dimensions provide a useful framework for describing the size and shape of objects, they offer only a limited perspective on the full range of properties and characteristics that define the reality of what we observe. To gain a more comprehensive understanding, we must consider additional dimensions related to time, energy, motion, and other fundamental aspects of the physical world. This broader perspective allows us to appreciate the richness and complexity of the universe in its entirety.
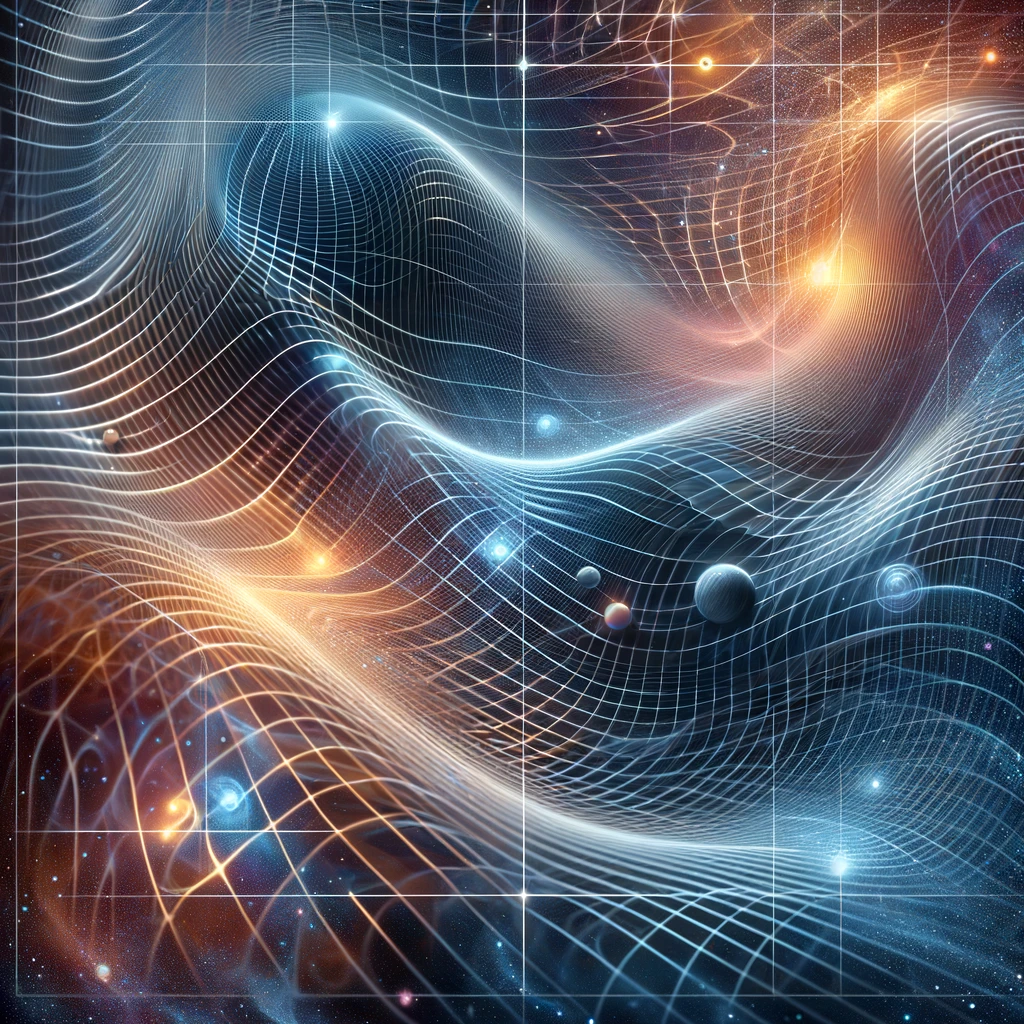
The concept of the fourth dimension can be approached from different perspectives, depending on the context in which it’s used. Here’s a general definition: In mathematics and physics, the fourth dimension refers to a theoretical extension beyond the familiar three dimensions of length, width, and height. While we experience and perceive the world in three spatial dimensions, the concept of the fourth dimension allows us to explore additional dimensions beyond our direct sensory experience. There are four basic categories that can be used to group different approaches of the fourth dimension:
Spatial Dimensions: In geometry and physics, spatial dimensions are used to describe the position and movement of objects in space. The first three dimensions—length, width, and height—define the physical extent of objects in three-dimensional space. The fourth dimension, when considered spatially, is typically conceptualized as a direction orthogonal to the three familiar dimensions.
Time as the Fourth Dimension: In the theory of relativity developed by Albert Einstein, time is considered the fourth dimension alongside the three spatial dimensions. In this framework, known as spacetime, space and time are interconnected, forming a four-dimensional continuum. Objects and events in the universe are described not only by their positions in space but also by their positions in time.
Mathematical Dimensions: In mathematics, dimensions are used to describe the number of independent coordinates needed to specify a point in a space. For example, a point in two-dimensional space requires two coordinates (x, y), while a point in three-dimensional space requires three coordinates (x, y, z). The concept of higher-dimensional spaces, including the fourth dimension and beyond, is used in various mathematical theories, such as vector spaces and differential geometry.
Metaphorical Interpretations: Beyond its mathematical and physical meanings, the fourth dimension is often used metaphorically to describe abstract concepts or realms beyond ordinary experience. For example, in philosophical or spiritual contexts, the fourth dimension may refer to higher states of consciousness, alternate realities, or dimensions of existence beyond the physical world.
In summary, the fourth dimension can be understood in various ways depending on the context, ranging from a mathematical extension beyond three-dimensional space to the interconnectedness of space and time in the theory of relativity, and even metaphorical interpretations beyond conventional understanding.
There's scientific evidence of the 4th dimension, but not 5th, 6th, 7th...
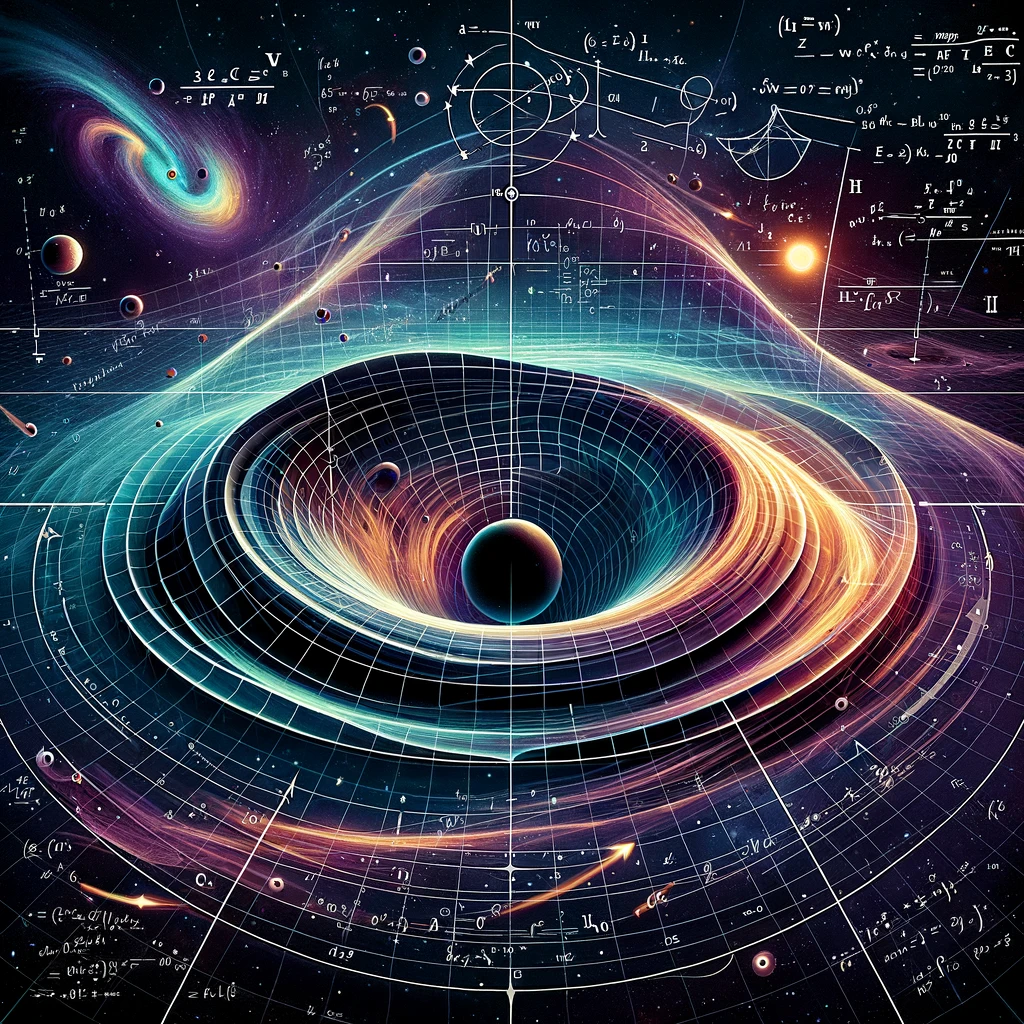
The concept of additional dimensions beyond the familiar three spatial dimensions (length, width, and height) and one temporal dimension (time) is a topic of theoretical speculation in certain branches of physics and mathematics. However, it’s important to clarify that the idea of higher dimensions (beyond the fourth) is primarily a theoretical construct and not directly substantiated by empirical evidence in the same way that the existence of the fourth dimension of time is supported by experimental observations and the principles of relativity.
In physics, the existence of a fourth dimension, time, is well-established and supported by experimental evidence from numerous phenomena, including time dilation in special and general relativity, as well as the behavior of particles in quantum mechanics.
The concept of higher dimensions beyond the fourth dimension arises in theoretical frameworks such as string theory, which suggests that the universe may have additional spatial dimensions beyond the three we perceive. However, these extra dimensions are typically hypothesized to be compactified or curled up at very small scales, making them inaccessible to direct observation with current technology.
While the idea of higher dimensions plays a crucial role in certain theoretical frameworks and mathematical models, such as string theory and Kaluza-Klein theory, there is currently no direct experimental evidence for the existence of dimensions beyond the familiar four. As such, the exploration of higher-dimensional spaces beyond the fourth dimension remains a topic of ongoing theoretical research and speculation, rather than established scientific fact.
Why a Systems Engineer should consider the 4th dimension
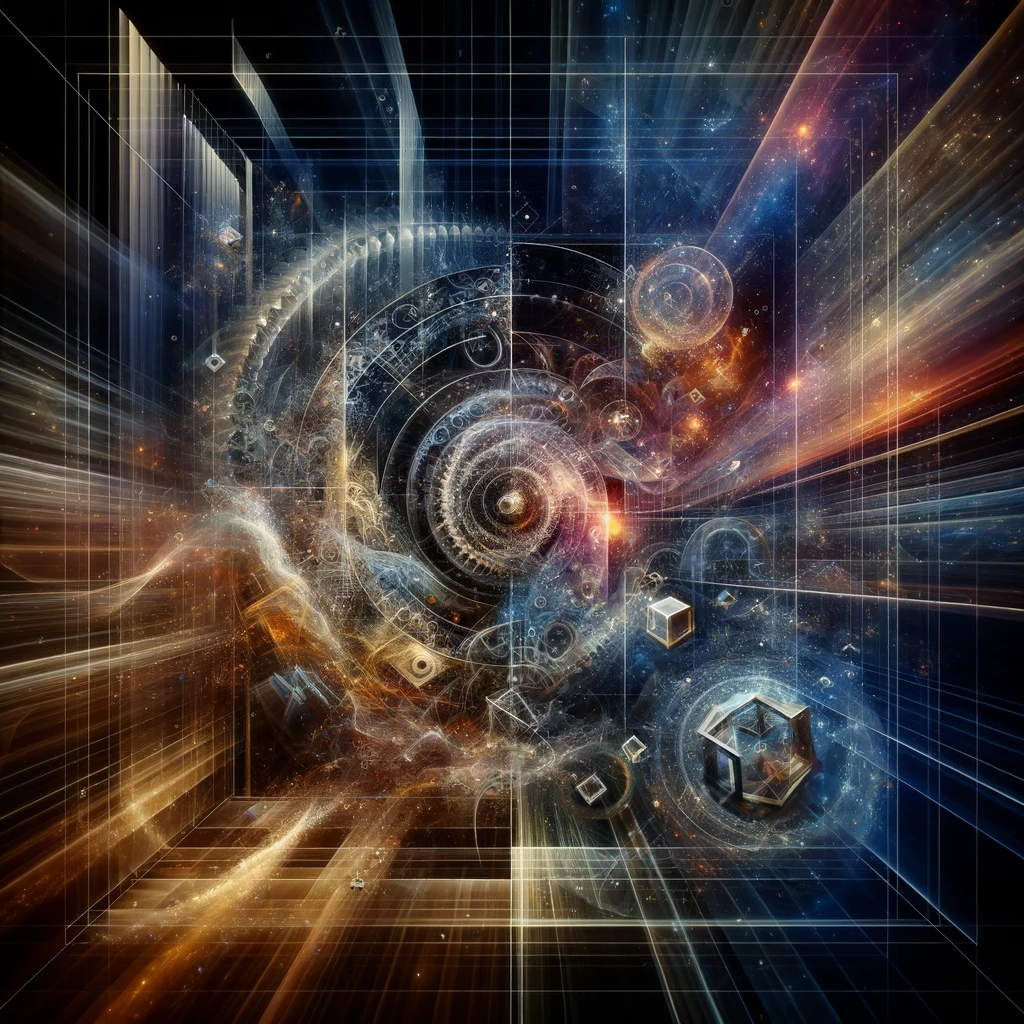
For a systems engineer, taking the “big picture” into account is essential for truly advancing in their field. This includes considering not only the immediate system they are working on but also understanding how it fits into the broader context of the environment in which it operates. Incorporating the concept of the fourth dimension, as well as various views and theories surrounding it, can provide valuable insights and perspectives that enhance the engineer’s ability to design, analyze, and optimize complex systems. Here’s how:
Temporal Considerations: The fourth dimension, time, is a critical factor in systems engineering. Understanding the temporal aspects of a system—such as its evolution over time, dynamic behavior, and response to changes—allows engineers to design more robust and adaptive systems. Considering time can help anticipate future needs, plan for scalability, and address potential challenges that may arise over the system’s lifecycle.
Complex Systems Dynamics: Many systems exhibit dynamic behaviors that unfold over time, influenced by various internal and external factors. By incorporating the fourth dimension into their analysis, systems engineers can better model and understand these dynamics, leading to more accurate predictions, improved control strategies, and enhanced system performance.
Multidimensional Views: Different views and theories of the fourth dimension, including those from physics, mathematics, and philosophy, offer diverse perspectives on the nature of reality and the structure of spacetime. Systems engineers can benefit from exploring these perspectives to broaden their understanding of complex systems and uncover new opportunities for innovation.
Holistic System Design: Taking a holistic approach to system design involves considering not only the system components and their interactions but also their broader context and impact. By embracing multidimensional views of the fourth dimension, engineers can develop more holistic designs that account for interconnectedness, interdependencies, and emergent properties, leading to more resilient and adaptable systems.
Innovative Problem-solving: Engaging with different views and theories of the fourth dimension fosters creativity and innovation in problem-solving. By challenging conventional assumptions and exploring alternative perspectives, engineers can discover novel solutions to complex challenges and push the boundaries of what is possible in systems design and engineering.
In summary, incorporating the concept of the fourth dimension and embracing diverse views and theories surrounding it can enrich the practice of systems engineering. By taking the “big picture” into account, engineers can develop more comprehensive, resilient, and innovative solutions that advance the field and address the complex challenges of our interconnected world.
The Vantage Point is an astronomical view that's all-encompassing of the 4th dimension
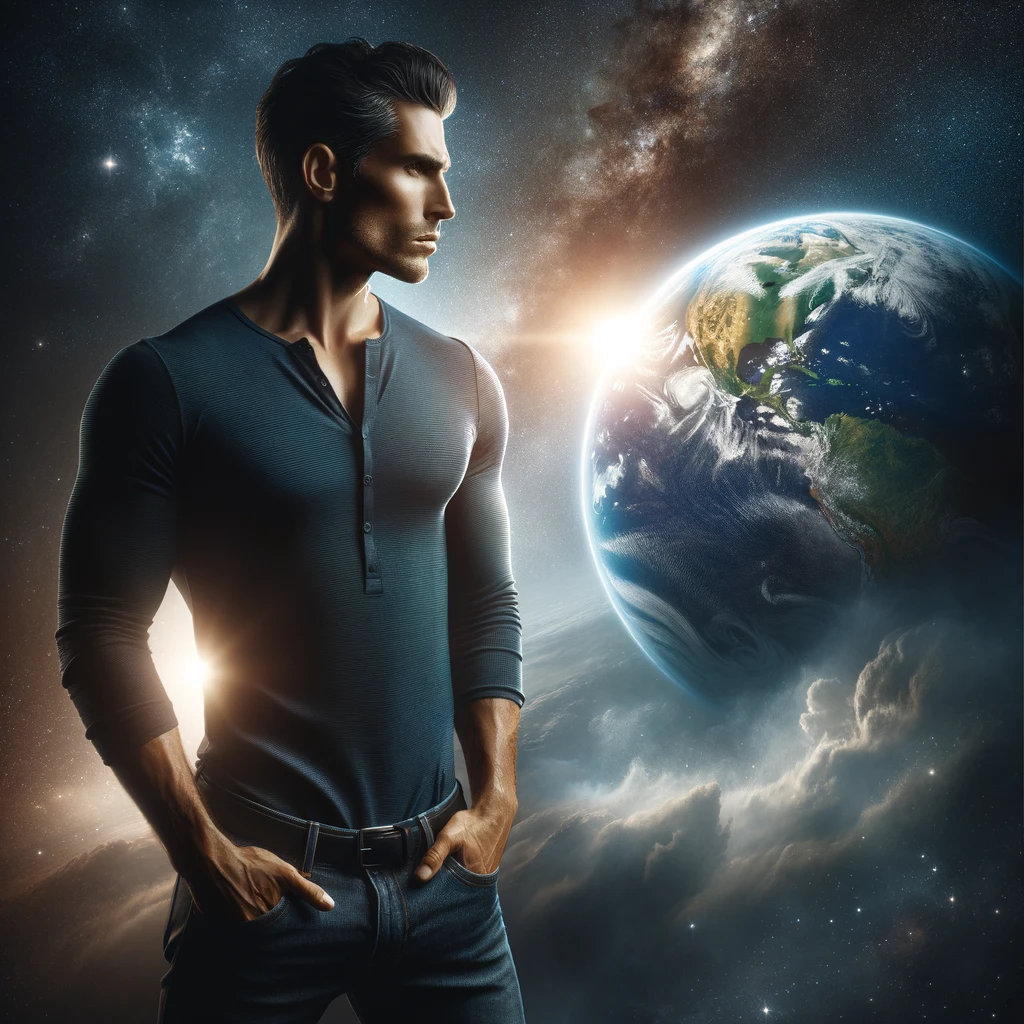
A skilled systems engineer employs the best critical and creative thinking skills possible to ensure that nothing slips past them, because in the end, they do not want to harbor regret, not in their profession, not in their SOI… does anyone want to harbor regret over anything in life? In this timeless zone of the XSE vantage point, you have the ability to explore the fourth dimension (which many physicists and mathematicians specify as time, space, etc…). Step back, outside of time, outside of your own life (as reality goes beyond this moment and your life) as if it’s already over. Everyone eventually learns something that they did not previously know. Time is ticking, and once time goes, it’s gone, and then each one realizes individually how short it all was.
Spatial, Temporal, Mathematical and Metaphorical Dimensions
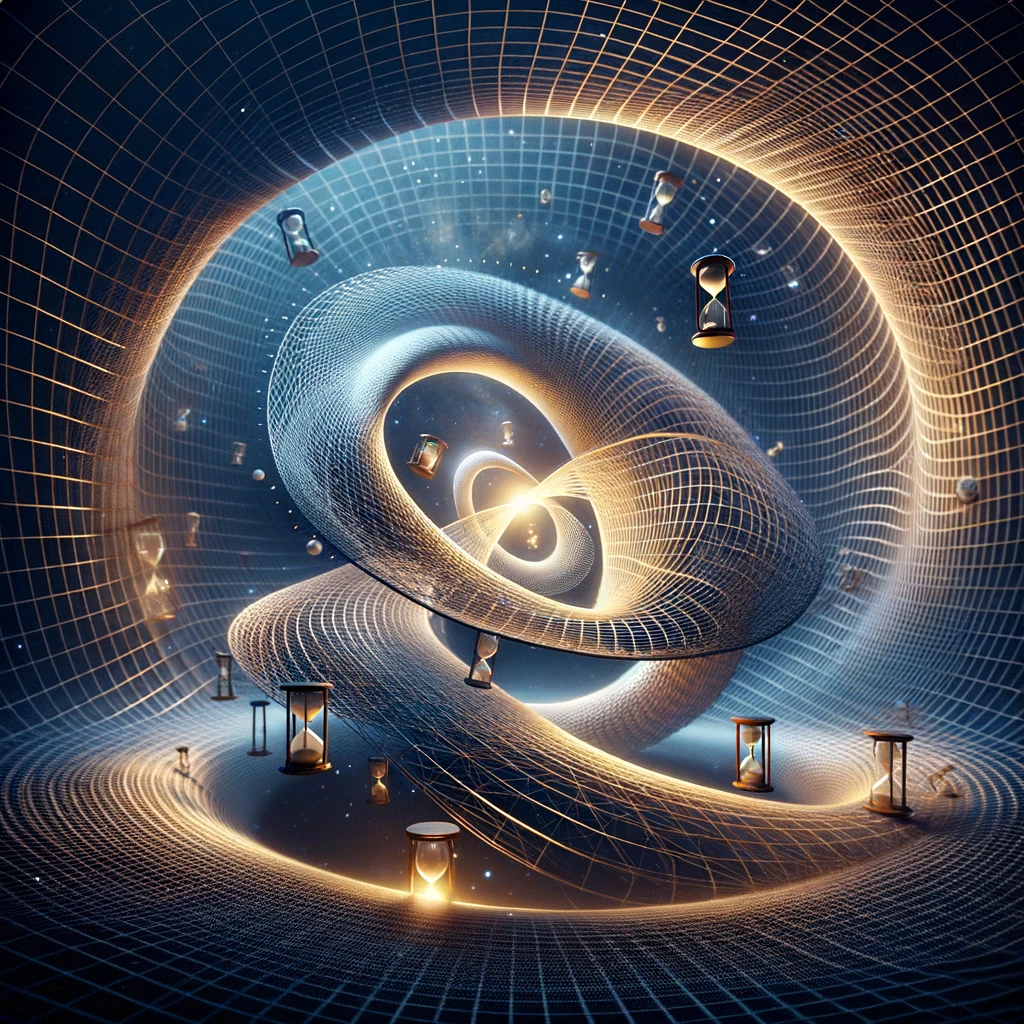
Exploring the concept of the fourth dimension can significantly enhance a systems engineer’s excellence by providing valuable insights and perspectives that extend beyond conventional three-dimensional thinking. Here’s how:
Enhanced Visualization: Understanding the concept of the fourth dimension challenges engineers to think beyond the limitations of three-dimensional space. By visualizing systems and processes in higher-dimensional spaces, engineers can gain a deeper understanding of complex relationships, interactions, and dependencies that may not be immediately apparent in three dimensions.
Complex Systems Modeling: Many real-world systems exhibit behaviors that are inherently multidimensional and dynamic. By incorporating the concept of the fourth dimension into their modeling and simulation approaches, engineers can develop more accurate representations of system dynamics, enabling better prediction, analysis, and optimization of system performance.
Temporal Analysis: In addition to spatial dimensions, the fourth dimension—time—is a critical factor in systems engineering. Exploring the temporal dimension allows engineers to analyze system behavior over time, identify trends and patterns, and anticipate future states. This temporal perspective is essential for designing systems that are robust, adaptable, and resilient to change.
Holistic System Design: The concept of the fourth dimension encourages systems engineers to take a holistic approach to system design, considering not only the physical components and their spatial relationships but also their temporal evolution and broader context. By embracing multidimensional thinking, engineers can develop more comprehensive and integrated solutions that address the complex interplay of factors affecting system performance.
Innovative Problem-solving: Exploring the fourth dimension fosters creativity and innovation in problem-solving. By thinking “outside the box” and considering alternative perspectives, engineers can discover novel solutions to challenging problems and unlock new opportunities for improvement and advancement in systems design and engineering.
Interdisciplinary Insights: The concept of the fourth dimension bridges the gap between mathematics, physics, and engineering, providing engineers with insights from diverse disciplines. By drawing on principles from fields such as topology, geometry, and relativity, engineers can enrich their understanding of complex systems and develop innovative approaches to system design and analysis.
In summary, exploring the concept of the fourth dimension in relation to systems engineering can broaden engineers’ perspectives, enhance their analytical capabilities, and inspire creative solutions to complex challenges. By embracing multidimensional thinking, engineers can achieve excellence in designing, analyzing, and optimizing systems for a wide range of applications.
The Fourth Dimension as Space
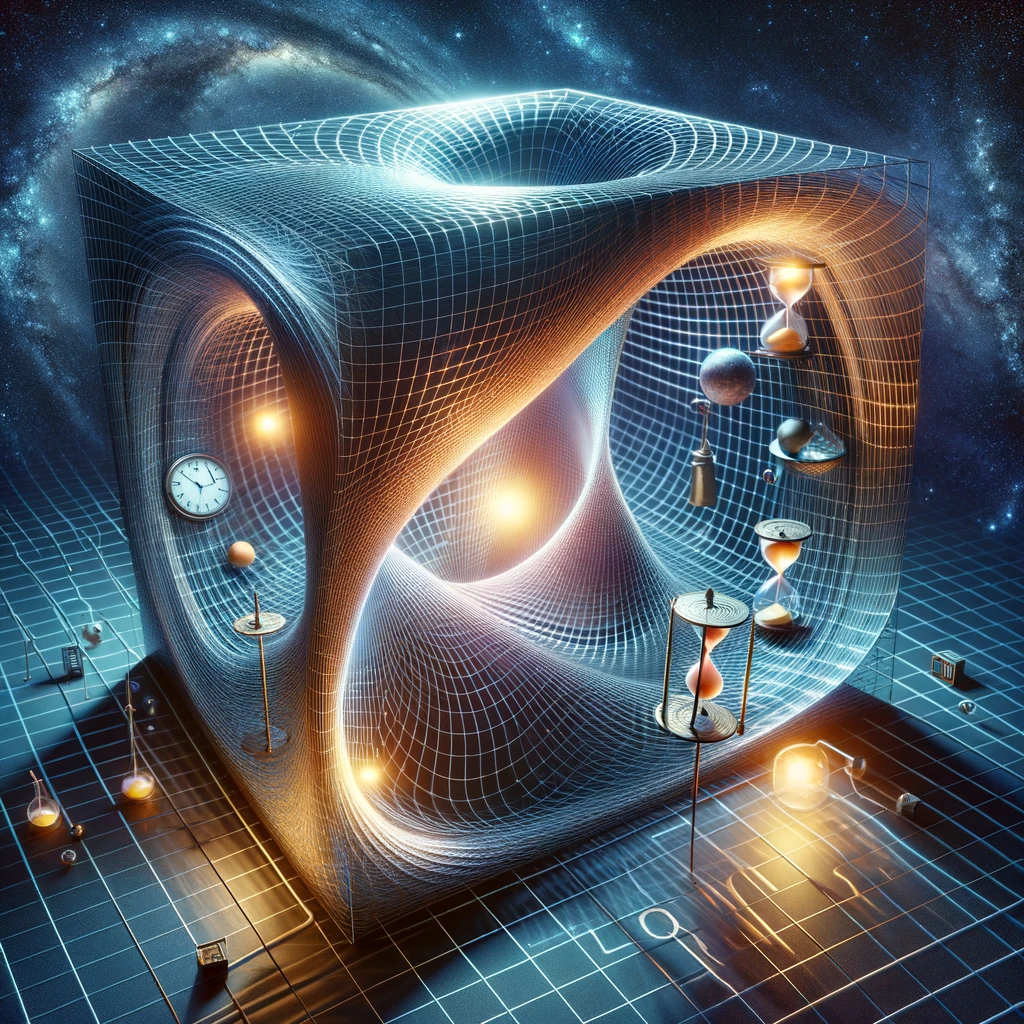
Exploring the concept of the fourth dimension in relation to spatial dimensions can offer several benefits to a systems engineer:
Expanded Spatial Understanding: By considering the fourth dimension as a spatial direction orthogonal to the familiar three dimensions, engineers can expand their spatial understanding beyond conventional three-dimensional thinking. This broader perspective allows engineers to conceptualize spatial relationships and configurations that may not be immediately apparent in three dimensions alone.
Advanced Visualization Techniques: Understanding the fourth spatial dimension enables engineers to develop advanced visualization techniques for representing complex spatial data and configurations. By visualizing systems in higher-dimensional spaces, engineers can gain insights into intricate spatial patterns, structures, and interactions, facilitating better analysis and decision-making.
Optimization of Spatial Layouts: Incorporating the fourth spatial dimension into spatial layout and design processes can lead to more optimized and efficient solutions. Engineers can explore alternative spatial configurations and arrangements that leverage the additional spatial dimension to minimize resource usage, improve accessibility, and enhance system performance.
Novel Approaches to Spatial Analysis: Exploring the fourth spatial dimension opens up new possibilities for spatial analysis techniques and methodologies. Engineers can develop innovative algorithms and tools for analyzing spatial data, identifying spatial patterns, and optimizing spatial arrangements based on higher-dimensional considerations.
Improved System Integration and Compatibility: Considering the fourth spatial dimension allows engineers to address challenges related to system integration and compatibility in complex spatial environments. By accounting for additional spatial dimensions, engineers can design systems that are more compatible with diverse spatial contexts and exhibit greater flexibility and adaptability in varying spatial conditions.
Enhanced Spatial Planning and Design: Incorporating the fourth spatial dimension into spatial planning and design processes enables engineers to develop more holistic and forward-thinking solutions. By considering how systems evolve and interact over time in higher-dimensional spatial spaces, engineers can anticipate future spatial requirements, mitigate spatial constraints, and design systems that are more resilient to spatial changes and fluctuations.
In summary, exploring the concept of the fourth dimension in relation to spatial dimensions offers systems engineers a powerful framework for expanding their spatial understanding, optimizing spatial layouts, developing novel spatial analysis techniques, and designing more integrated and adaptable systems in complex spatial environments.
Time as the Fourth Dimension
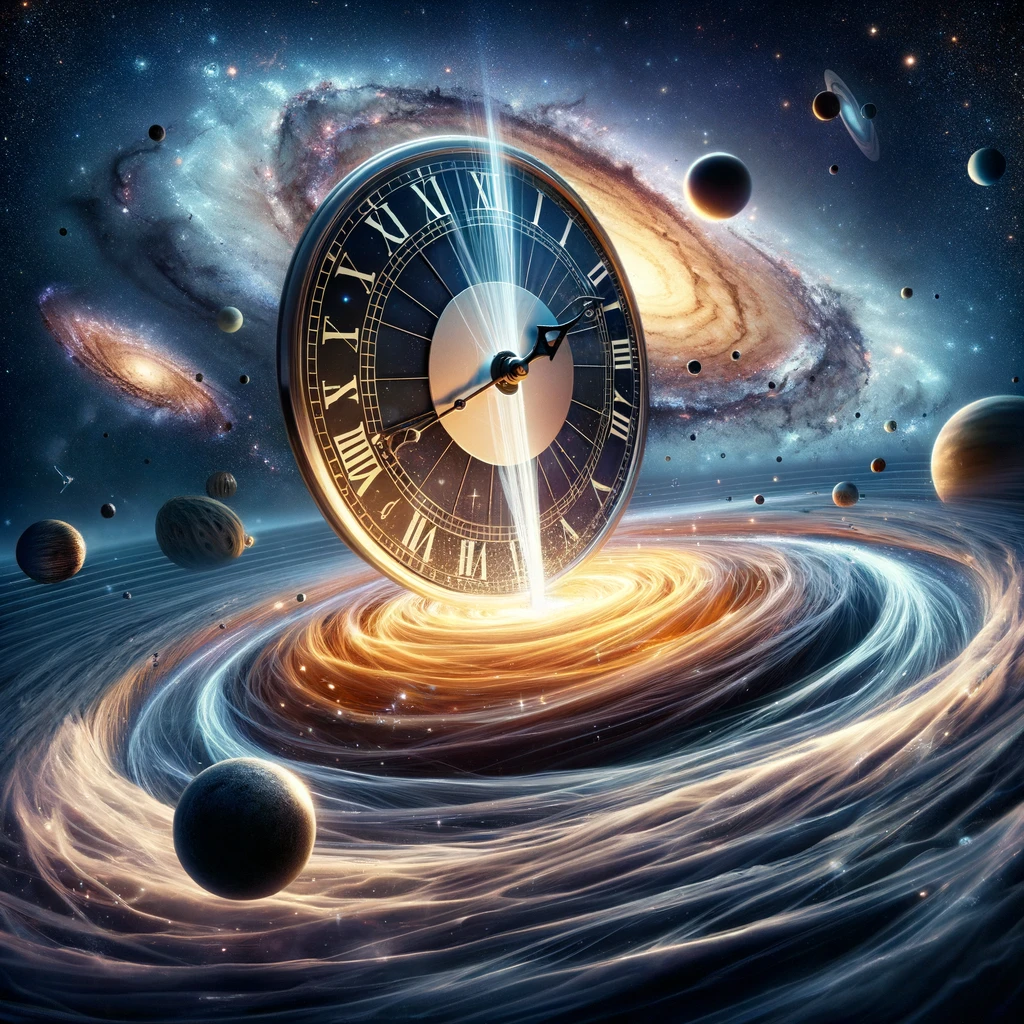
Exploring the concept of time as the fourth dimension, as described in Einstein’s theory of relativity, can provide several benefits to a systems engineer:
Temporal Analysis and Prediction: Viewing time as the fourth dimension allows engineers to analyze systems dynamically over time. By considering how systems evolve and change over temporal intervals, engineers can make better predictions about future system behavior, identify trends, and anticipate potential issues or failures.
System Dynamics Modeling: Incorporating time as a dimension in system modeling enables engineers to develop more comprehensive models that capture the dynamic behavior of systems. By representing the temporal evolution of system components and interactions, engineers can simulate system dynamics, assess performance under different scenarios, and optimize system behavior over time.
Temporal Synchronization and Coordination: Understanding time as a dimension in system design allows engineers to synchronize and coordinate system components and processes more effectively. By accounting for temporal dependencies and constraints, engineers can ensure that system operations are properly sequenced, timed, and coordinated to achieve desired outcomes and avoid conflicts or bottlenecks.
Temporal Control Strategies: Viewing time as an integral part of system design enables engineers to develop sophisticated control strategies that account for temporal considerations. By incorporating feedback mechanisms and temporal logic into control algorithms, engineers can regulate system behavior, respond to changing conditions over time, and adaptively adjust system parameters to achieve desired performance objectives.
Temporal Planning and Resource Allocation: Incorporating time as a dimension in planning and resource allocation processes allows engineers to optimize system performance over time. By considering temporal constraints, deadlines, and resource availability, engineers can schedule activities, allocate resources, and prioritize tasks to maximize efficiency, minimize delays, and meet project timelines.
Temporal Resilience and Adaptability: Recognizing time as a dimension in system design enables engineers to develop systems that are resilient and adaptable to temporal changes and disruptions. By designing systems with built-in flexibility and responsiveness to temporal fluctuations, engineers can mitigate the impact of unexpected events, recover from failures, and maintain system performance over time.
In summary, exploring time as the fourth dimension in the context of systems engineering provides engineers with valuable insights into system dynamics, temporal dependencies, and performance optimization strategies. By incorporating temporal considerations into system design, modeling, control, and planning processes, engineers can develop more robust, efficient, and adaptable systems that meet evolving needs and challenges over time.
Spacetime as a concept of the Fourth Dimension
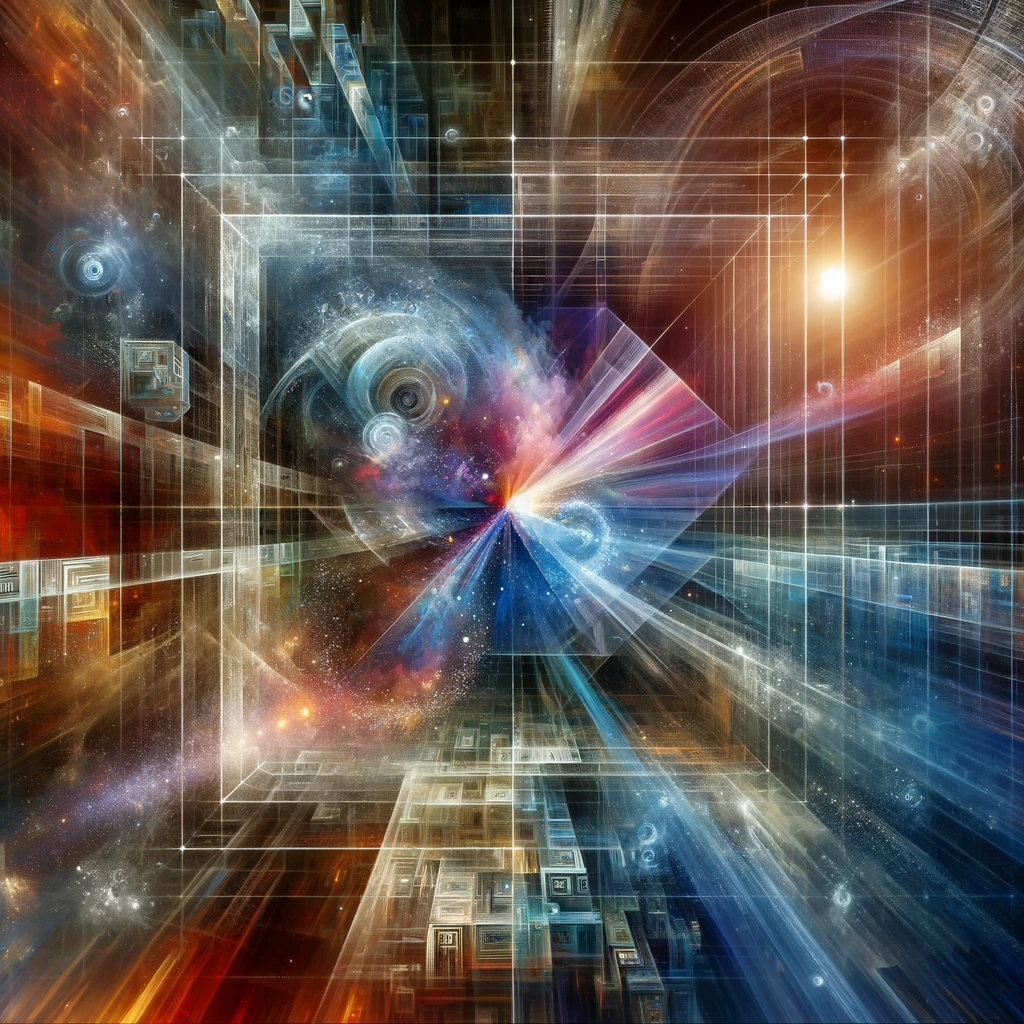
In a sense Spacetime and the Fourth Dimension are the same, but it’s important to clarify the distinction between the two concepts:
Spacetime: Spacetime is a unified framework that combines the three spatial dimensions (length, width, and height) with the dimension of time into a single, four-dimensional continuum. In this framework, objects and events are described not only by their positions in space but also by their positions in time. Spacetime is a fundamental concept in physics, particularly in Einstein’s theory of relativity, where it serves as the stage on which the dynamics of the universe unfold.
Fourth Dimension: The term “fourth dimension” is often used in a more general sense to refer to an additional spatial dimension beyond the three familiar dimensions. While spacetime does encompass four dimensions—three spatial dimensions and one dimension of time—when people talk about the “fourth dimension” outside of the context of spacetime, they may be referring to a hypothetical spatial dimension beyond our ordinary perception.
So, while spacetime incorporates the fourth dimension in the context of time, the term “fourth dimension” is sometimes used more broadly to explore speculative ideas about additional spatial dimensions beyond the three we experience in everyday life.
Mathematical Fourth Dimension
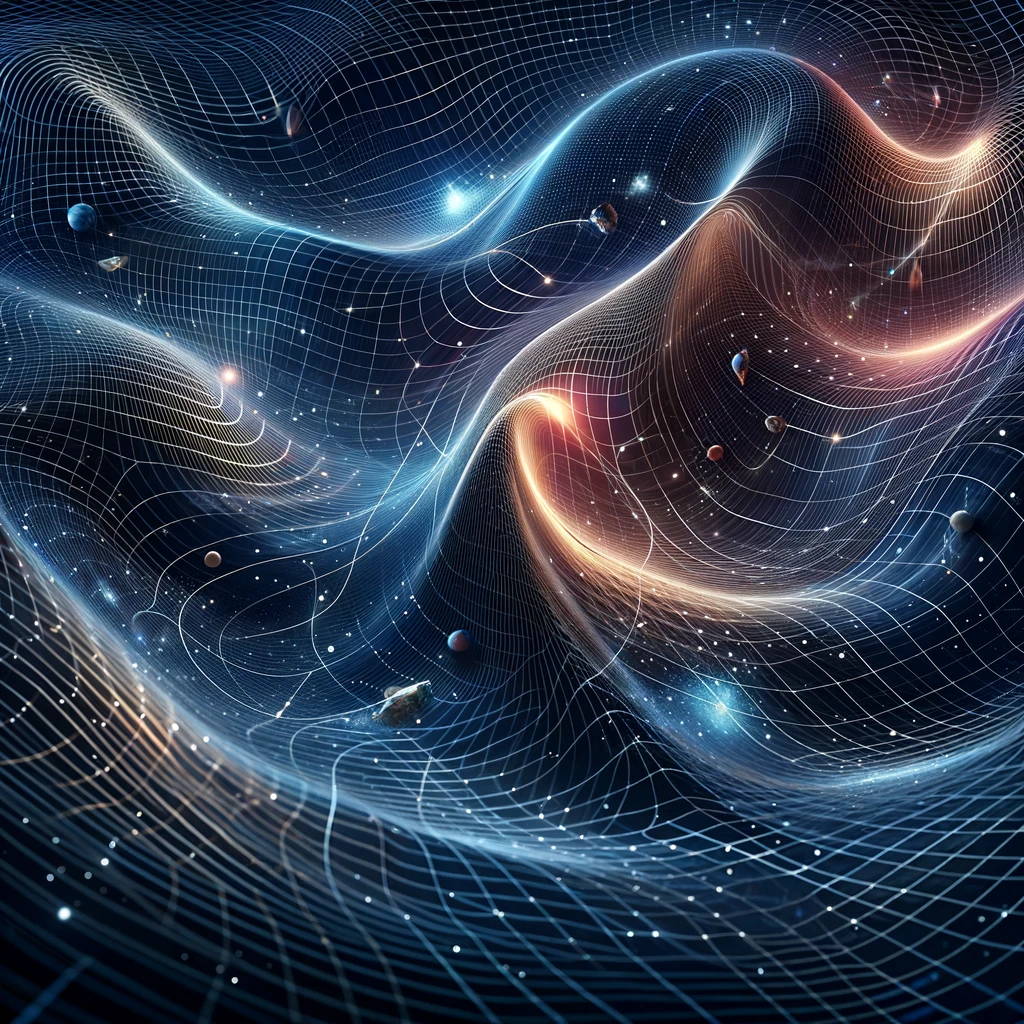
Exploring the concept of higher-dimensional spaces, including the fourth dimension and beyond, in relation to mathematical dimensions can provide several benefits to a systems engineer:
Advanced Data Representation: Higher-dimensional spaces offer engineers a more sophisticated framework for representing complex data and system states. By leveraging additional dimensions, engineers can create richer, more detailed representations of system components, interactions, and relationships, enabling more accurate analysis and modeling.
Multidimensional Analysis Techniques: Understanding higher-dimensional spaces allows engineers to develop advanced analysis techniques for studying system behavior and performance. By applying mathematical tools from fields such as linear algebra and differential geometry, engineers can analyze multidimensional data sets, identify patterns, and extract valuable insights to inform system design and optimization.
Optimization in Multidimensional Spaces: Exploring higher-dimensional spaces enables engineers to optimize system performance across multiple dimensions simultaneously. By considering a broader range of factors and constraints in multidimensional optimization problems, engineers can identify more efficient solutions, optimize resource allocation, and improve overall system effectiveness.
Enhanced System Visualization: Visualizing higher-dimensional spaces challenges engineers to develop innovative visualization techniques for representing multidimensional data and system configurations. By visualizing system states in higher-dimensional spaces, engineers can gain new perspectives on system structure, dynamics, and interactions, facilitating better understanding and decision-making.
Robustness to Dimensionality Reduction: Recognizing the potential complexity of higher-dimensional spaces prepares engineers to address challenges related to dimensionality reduction in real-world systems. By understanding the trade-offs involved in reducing the dimensionality of data and models, engineers can develop robust techniques for simplifying complex systems while preserving essential information and relationships.
Interdisciplinary Insights: Exploring higher-dimensional spaces fosters interdisciplinary collaboration and cross-pollination of ideas between mathematics, engineering, and other fields. By drawing on mathematical theories of higher-dimensional spaces, engineers can leverage insights and techniques from diverse disciplines to tackle complex systems engineering challenges more effectively.
In summary, exploring higher-dimensional spaces in relation to mathematical dimensions provides systems engineers with valuable tools and perspectives for representing, analyzing, and optimizing complex systems. By embracing the richness of higher-dimensional spaces, engineers can develop more sophisticated models, analysis techniques, and visualization methods that enhance their ability to design, analyze, and optimize systems across a wide range of applications.
The Metaphorical Fourth Dimension
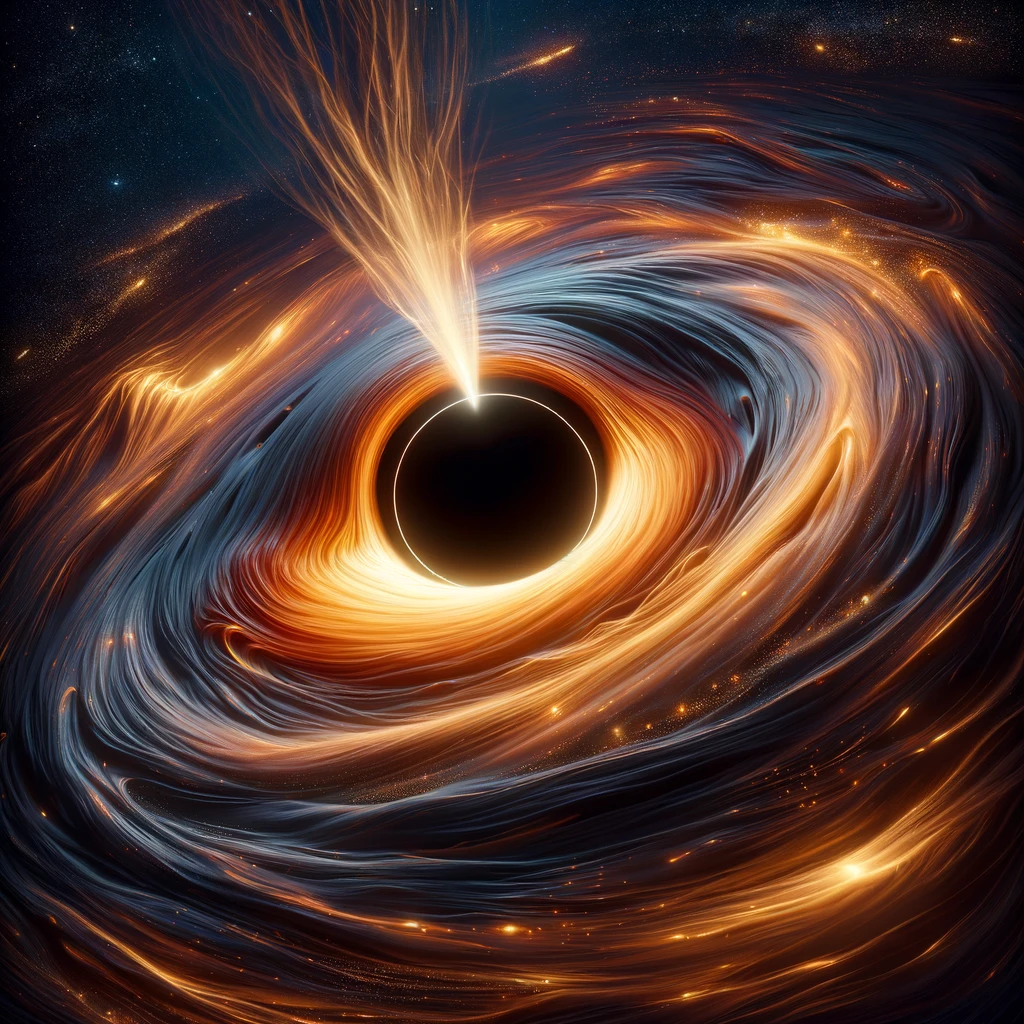
Exploring the metaphorical interpretations of the fourth dimension can provide several benefits to a systems engineer:
Expanded Problem-Solving Perspectives: Metaphorical interpretations of the fourth dimension encourage systems engineers to think beyond traditional boundaries and explore unconventional solutions to complex problems. By considering abstract concepts such as higher states of consciousness or reality beyond what meets the eye, engineers can unlock new perspectives and insights that inspire innovative approaches to system design and optimization.
Creative Solution Generation: Metaphorical interpretations of the fourth dimension stimulate creativity and imagination in problem-solving. By drawing inspiration from philosophical or spiritual concepts associated with the fourth dimension, engineers can generate novel ideas, concepts, and design principles that challenge conventional thinking and push the boundaries of what is possible in systems engineering.
Holistic Systems Thinking: Metaphorical interpretations of the fourth dimension encourage systems engineers to adopt a holistic approach to system design and analysis. By considering dimensions of existence beyond the physical world, engineers can develop systems that address not only functional requirements but also broader human needs, values, and aspirations, leading to more meaningful and impactful solutions.
Systems Integration and Harmony: Exploring metaphorical interpretations of the fourth dimension promotes systems integration and harmony by emphasizing interconnectedness, unity, and synergy. By viewing systems as part of larger wholes or interconnected networks of existence, engineers can design systems that resonate with their broader environment, promote sustainability, and contribute to greater harmony and balance in the world.
Ethical and Social Considerations: Metaphorical interpretations of the fourth dimension encourage systems engineers to consider ethical, social, and moral dimensions in their work. By reflecting on higher states of consciousness or alternate realities, engineers can develop systems that promote human well-being, social justice, and environmental sustainability, aligning with broader ethical principles and values.
Inspiration for Transdisciplinary Collaboration: Metaphorical interpretations of the fourth dimension inspire transdisciplinary collaboration and dialogue between systems engineering and other fields such as philosophy, psychology, spirituality, and the arts. By engaging with diverse perspectives and worldviews associated with the fourth dimension, engineers can enrich their understanding of complex systems and foster interdisciplinary collaborations that lead to more holistic and transformative solutions.
In summary, exploring metaphorical interpretations of the fourth dimension provides systems engineers with opportunities to expand their problem-solving perspectives, stimulate creativity and imagination, foster holistic systems thinking, promote ethical and social considerations, and inspire transdisciplinary collaboration. By embracing the richness of metaphorical interpretations, engineers can develop systems that are not only technically sound but also deeply meaningful, purposeful, and transformative in addressing the complex challenges of our time.
In a metaphorical sense, the idea of the “fourth dimension” representing the “inside” or what lies beneath the surface of a substance can be intriguing. While traditionally, the concept of the fourth dimension often refers to time in physics or mathematics, when applied metaphorically to understanding substances or entities, it can imply exploring deeper layers or hidden aspects beyond what is immediately visible or tangible.
Here’s how the metaphorical interpretation could be articulated:
“The Fourth Dimension: Delving beyond the surface, the metaphorical ‘fourth dimension’ unveils the inner intricacies and hidden depths residing within the substance. Just as the three spatial dimensions define the external form and structure, the fourth dimension offers insight into the unseen realms beneath the surface. Within this dimension lie the underlying properties, compositions, and dynamics that shape the substance’s essence. It serves as a gateway to explore the profound intricacies and complexities concealed within, transcending mere surface appearances to reveal the substance’s true nature and potential. Thus, the fourth dimension invites exploration into the depths, unlocking a deeper understanding and appreciation of the substance’s inherent richness and complexity.”
In engineering, the substance that a subject is made of is typically referred to as the “material.” Engineers often analyze and work with various materials to design and construct structures, components, and systems for a wide range of applications. These materials can include metals, polymers, ceramics, composites, and more, each with its own unique properties, characteristics, and behaviors under different conditions. Understanding the properties and behavior of materials is essential in engineering for selecting appropriate materials for specific applications, ensuring structural integrity, and optimizing performance and durability.