Integrating Human Biology with the Internet of Things
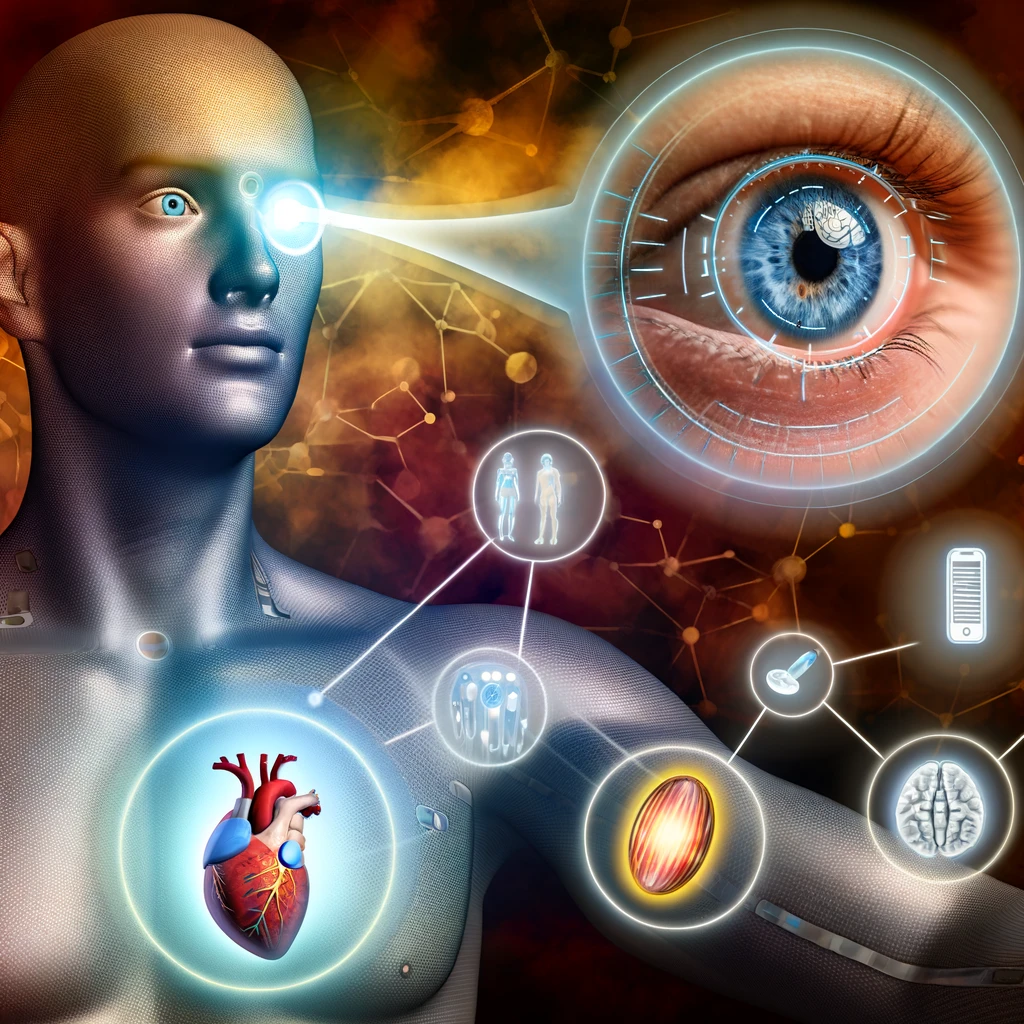
The integration of human biology with Internet of Things (IoT) technology is often referred to as “bio-IoT” or “biomedical IoT.” This concept involves the convergence of biological sensors, medical devices, and wearable technologies with IoT infrastructure to monitor, analyze, and manage various aspects of human health and well-being in real-time.
Bio-IoT systems typically involve the use of sensors and devices capable of capturing physiological data such as heart rate, blood pressure, temperature, glucose levels, and activity levels. These devices are often wearable or implantable and are connected to IoT networks, allowing for continuous data collection and transmission to centralized platforms for analysis.
The integration of human biology with IoT technology enables a wide range of applications in healthcare, including remote patient monitoring, personalized medicine, disease management, and preventive care. It allows healthcare providers to monitor patients’ health status more effectively, identify early warning signs of health problems, and intervene.
Overall, bio-IoT represents an area at the intersection of healthcare and technology, with the potential to revolutionize how people monitor and manage human health in the digital age.
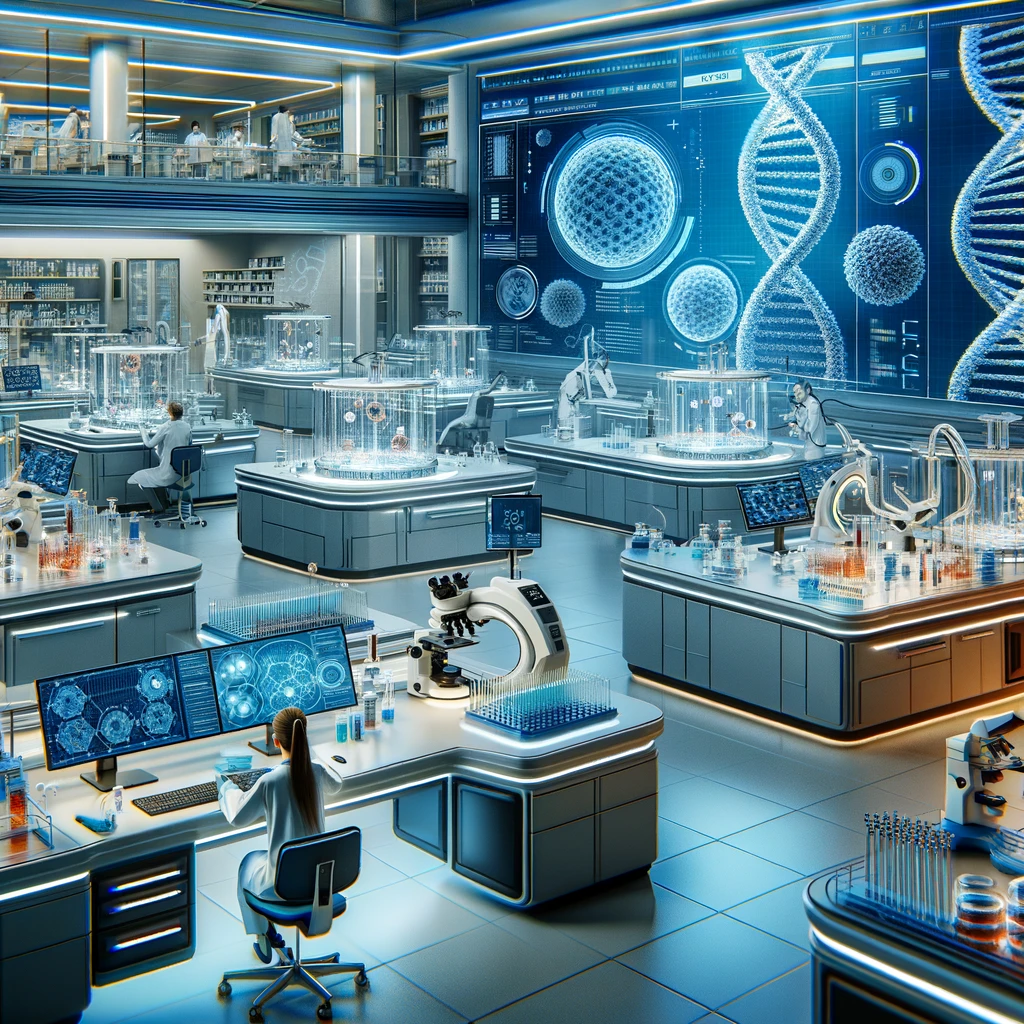
Here are direct sources from studies on the integration of IoT technology with human biology, highlighting developments in healthcare:
Edge Intelligence and Internet of Things in Healthcare: Amin, S., & Hossain, M. S. (2021). This study discusses sophisticated smart healthcare frameworks enabled by 5G and smart IoT sensors alongside edge computing, providing intelligent, real-time healthcare solutions. The paper addresses the evolution of edge computing architectures for healthcare and the application of artificial intelligence for health data classification and vital signs monitoring. Read more.
The Internet of Bio-Nano Things: Akyildiz, I. F., Pierobon, M., Balasubramaniam, S., & Koucheryavy, Y. (2015). This paper introduces the Internet of Bio-Nano Things (IoBNT), based on the interconnection of nanoscale devices and biological cells. It proposes a novel paradigm that could enable applications such as intra-body sensing networks and environmental control. Read more.
PANACEA: An Internet of Bio-NanoThings Application for Early Detection and Mitigation of Infectious Diseases: Akyildiz, I. F., et al. (2020). This study presents PANACEA, an IoBNT application focusing on the diagnosis and therapy of infectious diseases, showcasing a system that combines sensing, actuation, and computing processes for a responsive disease detection and infection recovery system. Read more.
IoT Middleware: A Survey on Issues and Enabling Technologies: Ngu, A. H., et al. (2017). The paper discusses the role of middleware in IoT systems, particularly for healthcare applications, and how it acts as an intermediary between IoT devices and applications to facilitate data processing and analysis. Read more.
A Systematic Review of Bio-Cyber Interface Technologies and Security Issues for Internet of Bio-Nano Things: Zafar, S., et al. (2021). This comprehensive review focuses on bio-cyber interface technologies essential for IoBNT, addressing the integration of biological and cyber systems and highlighting security and privacy considerations. Read more.
These sources provide insights into the latest research and development efforts at the intersection of IoT technology and human biology, exploring innovative healthcare solutions, the potential of bio-nano communications, and the challenges and opportunities in creating a more integrated and responsive healthcare ecosystem.
Commonly Implemented Bio-IoT Devices
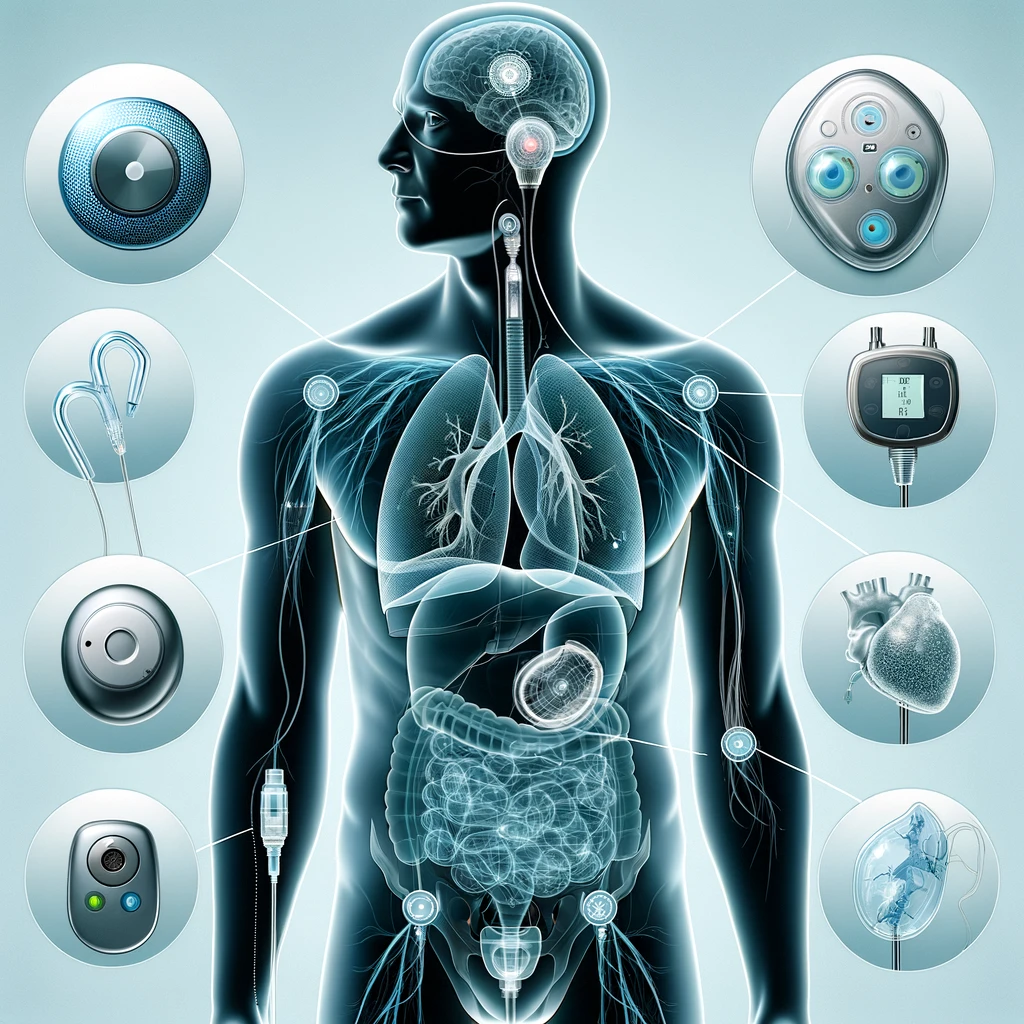
The adoption of IoT capabilities in medical implants varies significantly depending on the type of implant, its intended function, the technology’s maturity, and the regulatory environment.
Types of Medical Implants with IoT Capabilities:
Cardiac Devices: Some of the most advanced uses of IoT in medical implants can be found in cardiac care. Devices like pacemakers and implantable cardioverter-defibrillators (ICDs) often include remote monitoring capabilities, allowing healthcare providers to monitor patients’ heart rhythms and device function remotely.
Glucose Monitors: Implantable continuous glucose monitoring systems for patients with diabetes can transmit data wirelessly, allowing for real-time glucose level monitoring and better diabetes management.
Orthopedic Implants: There is emerging interest in smart orthopedic implants that can monitor the health of the implant and surrounding tissue, though widespread IoT integration in these devices is still in the early stages.
Factors Influencing IoT Adoption in Medical Implants:
Regulatory Approval: Medical devices, especially those that are implantable, undergo rigorous regulatory scrutiny. Integrating IoT capabilities adds complexity to the approval process, potentially slowing down adoption.
Security and Privacy Concerns: The risk of data breaches and hacking is a significant concern for IoT-enabled medical implants. Ensuring the security and privacy of patient data is critical and challenging.
Cost and Accessibility: The development and deployment of IoT-enabled implants involve higher costs, which can affect their adoption and accessibility, especially in lower-income regions.
Technological Challenges: Ensuring reliable and continuous data transmission, battery life, and compatibility with existing medical infrastructure are technical hurdles that need to be addressed.
In summary, while the integration of IoT features into medical implants holds great promise for enhancing patient care through real-time monitoring and personalized medicine, widespread adoption across all types of mechanical-function implants is not yet a reality. The pace of integration is influenced by a complex interplay of technological, regulatory, and ethical considerations.
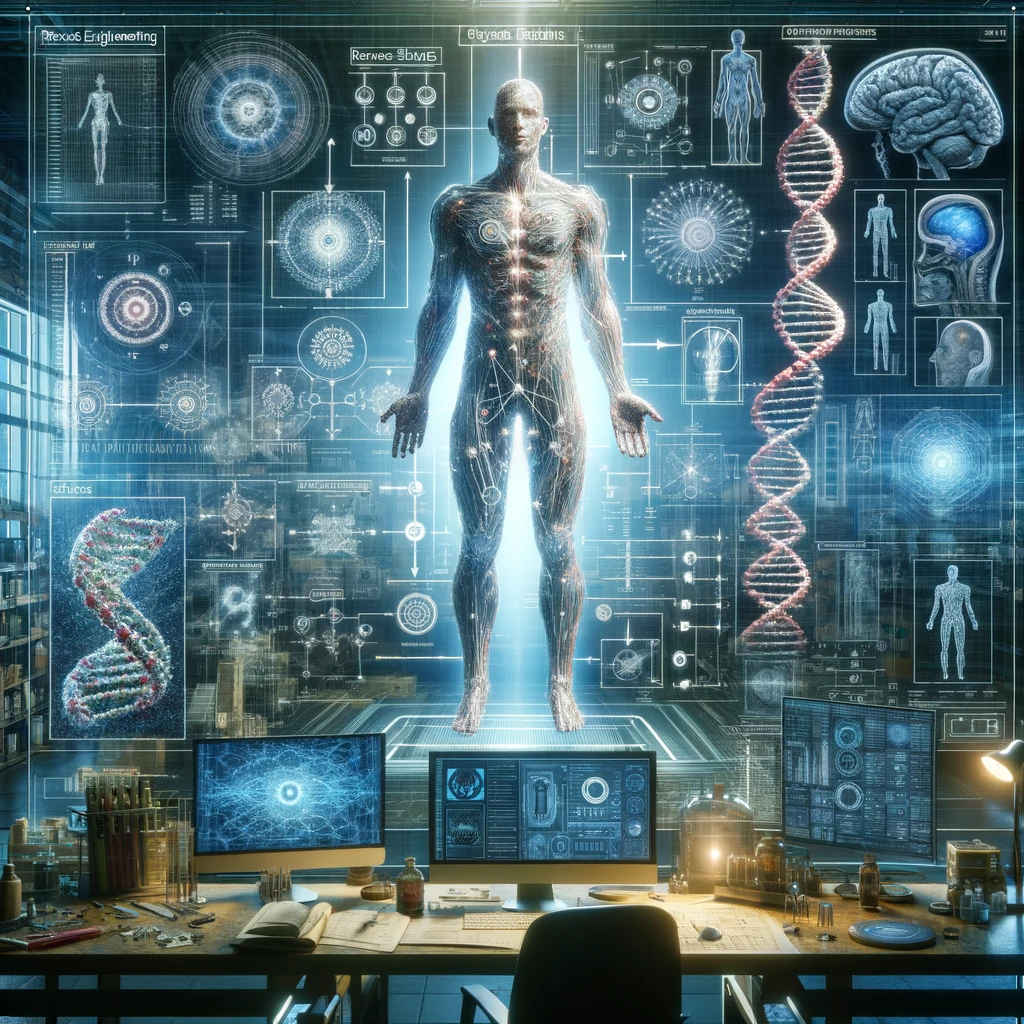
Pacemakers have evolved significantly since their inception, incorporating more advanced technology to improve patient care. In recent years, some pacemakers have indeed started to incorporate features that connect them to the Internet of Things (IoT), allowing for remote monitoring by healthcare providers. This advancement enables more efficient and real-time tracking of a patient’s status, potentially improving outcomes by allowing for quicker responses to any issues that may arise.
Key Benefits of IoT-Connected Pacemakers:
Remote Monitoring: Patients with IoT-connected pacemakers can be monitored remotely by healthcare professionals. This means that data on heart rhythms and the device’s performance can be continuously evaluated without the need for frequent in-person visits.
Early Detection of Complications: Any irregularities or malfunctions can be detected early, allowing for prompt intervention before a patient experiences significant health issues.
Convenience for Patients: Patients benefit from the convenience of not having to visit the hospital or clinic as often for routine pacemaker checks. This is particularly advantageous for those living in remote areas or with limited mobility.
Personalized Care: The detailed data collected can help healthcare providers tailor care more precisely to individual patient needs, potentially improving the effectiveness of treatment and management strategies.
Improved Patient Outcomes: By enabling more timely and personalized care, IoT-connected pacemakers may contribute to better overall patient outcomes and quality of life.
Considerations and Challenges:
Security and Privacy: The connection of medical devices to the internet raises significant concerns regarding data security and patient privacy. Ensuring the security of transmitted data is paramount to protect against unauthorized access and potential cyberattacks.
Regulatory and Ethical Issues: The use of IoT in healthcare devices like pacemakers involves navigating complex regulatory and ethical landscapes, ensuring compliance with medical standards and patient consent laws.
Technology Adoption: There is also the challenge of technology adoption among older patients who may not be as comfortable with digital and connected technologies.
In conclusion, while not all pacemakers are connected to the IoT, the trend towards incorporating such technology is growing, driven by the potential for improved monitoring and patient care. However, the successful implementation of these devices requires careful consideration of security, privacy, and regulatory issues.
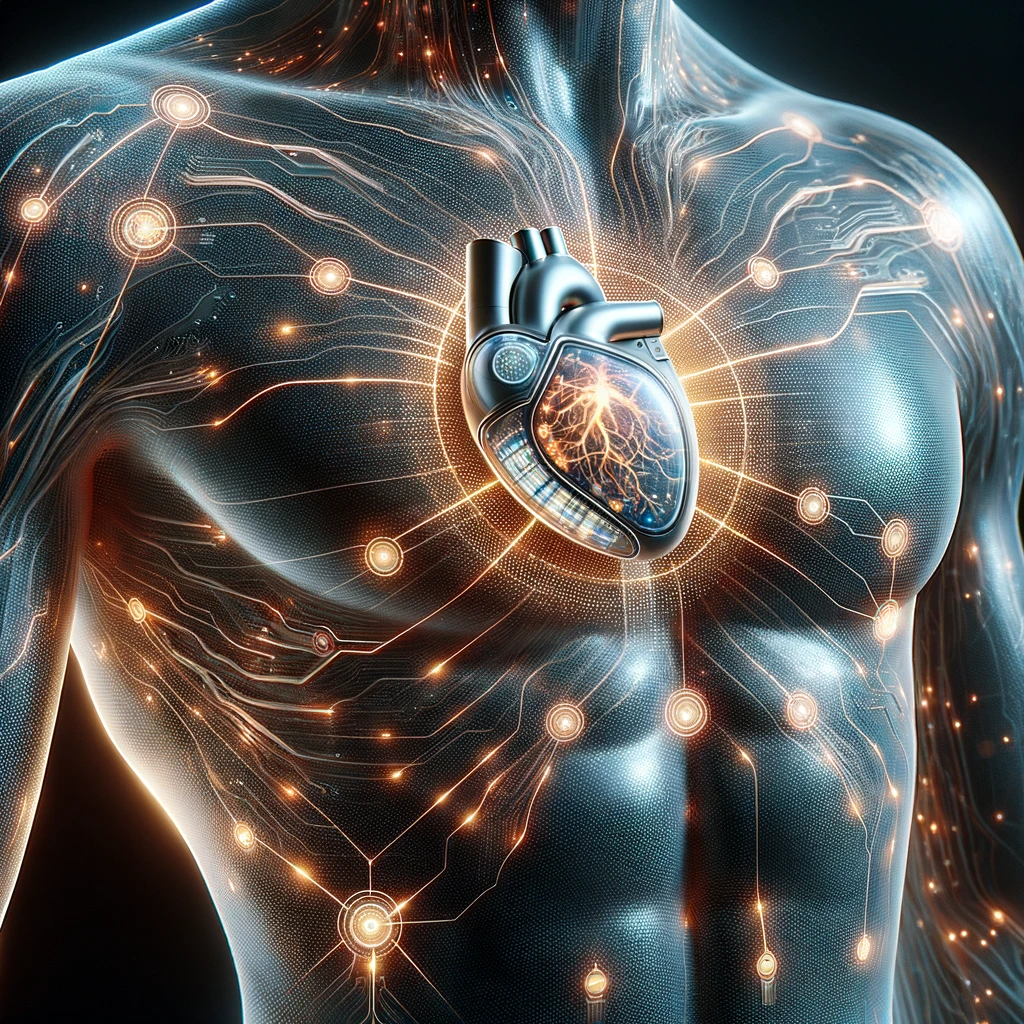
Here are more studies in the integration of IoT technology with human biology, reflecting the latest developments in healthcare and biomedical engineering:
Flexible and Stretchable Electronics for Biointegrated Devices: Kim, Ghaffari, Lu, and Rogers (2012) discuss advances in materials, mechanics, and manufacturing that enable the construction of high-quality electronics and optoelectronics. These can integrate with the soft, curvilinear surfaces of the human body, enhancing health monitoring, surgical procedures, and human-machine interfaces.
The Internet of Bio-Nano Things (IoBNT): Akyildiz, Pierobon, Balasubramaniam, and Koucheryavy (2015) introduce IoBNT, which involves the interconnection of nanoscale devices based on biological cells. This paradigm enables applications like intra-body sensing networks and environmental control, showcasing a novel approach to integrating biological components with technological networks.
Security and Privacy Techniques in IoT Environment: Lin and Yeh (2020) address the crucial aspects of security and privacy in the IoT environment, focusing on embedded systems, mobile devices, actuators, and sensors. This study underscores the need for robust security measures to protect data in IoT-based health monitoring systems.
IoT for Health Care: A Comprehensive Survey: Islam, Kwak, Kabir, Hossain, and Kwak (2015) review advances in IoT-based health care technologies. They examine network architectures/platforms, applications, and trends in IoT health care solutions, highlighting the importance of security and privacy features.
Tissue–Electronics Interfaces: Feiner and Dvir (2018) review advances in electronic devices that allow for data extraction and disease treatment by interfacing with organs and engineered tissues. This work highlights the potential for electronic systems to enhance medical treatments and health monitoring.
Biosensor and Chemosensor Concepts for IoT: Mayer and Baeumner (2019) discuss the integration of biosensor and chemosensor technologies with IoT, emphasizing their role in health monitoring, environmental control, and agricultural applications. This review highlights the challenges and future directions for sensor technologies in the IoT era.
sEMG Hand with IoT Sensors and Haptic Feedback: Li, Zhang, Sun, and Kong (2018) focus on integrating artificial sensory perception and haptic feedback in sEMG hands. This technology improves manipulation performance and perceptual embodiment for users, showcasing the potential of IoT sensors in prosthetic development.
Challenges and Technologies for Industrial IoT: Younan, Houssein, Elhoseny, and Ali (2020) provide a comprehensive review of challenges and recommended technologies for the Industrial Internet of Things (IIoT). They propose frameworks for data analysis and search in IoT, addressing the integration of ICT in IoT layers.
These advancements demonstrate the potential of IoT technology to revolutionize healthcare by enabling precise, real-time monitoring and treatment, while also addressing the challenges of security, privacy, and integration of biological and cyber systems.
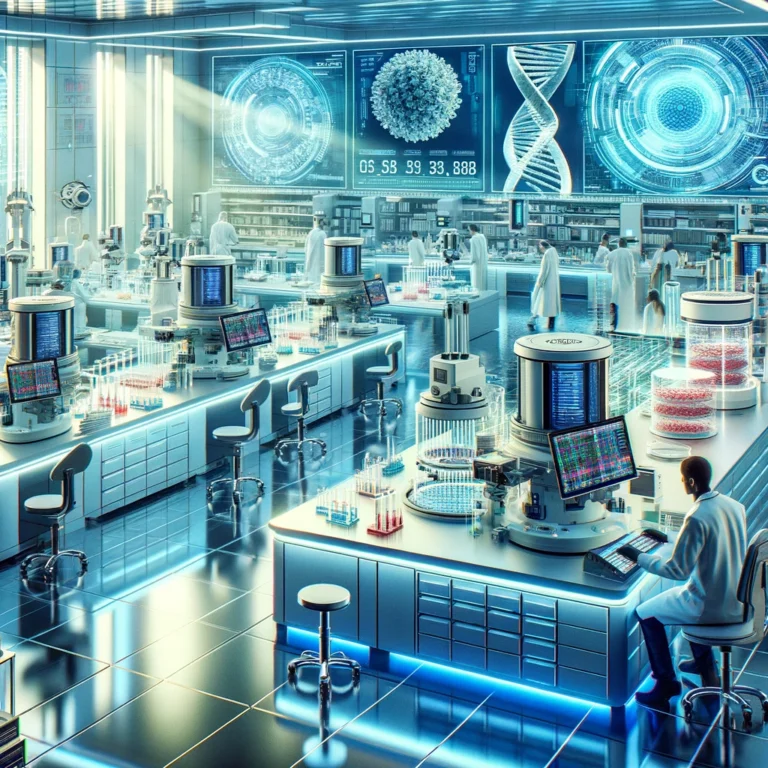
Neural Net, Chip, or Both? Here is what AI says:
The question of whether a neural net or a biochip is more likely to become the norm for connecting humans to the Internet of Things (IoT) involves considering current technological trends, limitations, and the specific applications of each technology. Both technologies have their unique strengths and could potentially work together rather than in opposition. Here’s a closer look at each and how they might interact:
Neural Networks
- Definition: In this context, a neural net (or neural network) typically refers to artificial neural networks, which are computing systems inspired by the biological neural networks of animal brains. These systems learn to perform tasks by considering examples, generally without being programmed with task-specific rules.
- Applications in IoT: Neural networks are primarily used for data analysis, pattern recognition, and decision-making processes within the IoT ecosystem. They can process and interpret the vast amounts of data generated by IoT devices, facilitating smarter decision-making and automation.
- Limitations and Strengths: While powerful for data processing and analysis, neural networks themselves do not connect humans directly to the IoT. They are, however, crucial for making sense of IoT data and enabling intelligent systems.
Biochips
- Definition: Biochips are miniaturized laboratories that can perform hundreds or thousands of simultaneous biochemical reactions. In the context of connecting humans to the IoT, biochips could be used to monitor health data or modify biological functions.
- Applications in IoT: Biochips have the potential to directly interface with the human body to collect health metrics or administer therapies, transmitting this data to IoT devices for monitoring, analysis, or remote medical consultations.
- Limitations and Strengths: Biochips can directly interface with biological systems, making them well-suited for medical applications and direct human integration with IoT. Their limitation lies in their current developmental stage, focusing more on health monitoring and less on broader IoT connectivity.
Integration and Future Trends
- Working Together: It’s likely that neural networks and biochips will work together rather than one becoming the norm over the other. Neural networks could process and analyze data collected by biochips implanted in or worn by humans, combining the strengths of both technologies. This integration could enable advanced health monitoring, personalized medicine, and even direct brain-to-computer interfaces.
- Future Developments: Research in both fields is rapidly advancing. Biochips are becoming more sophisticated, capable of monitoring a wider range of health indicators and even interacting with biological systems in real-time. Neural networks are becoming more efficient and capable of processing complex datasets, making them increasingly relevant for interpreting the data from biochips and other IoT devices.
In conclusion, the future is likely to see a convergence of technologies where neural networks and biochips complement each other, offering a more integrated and intelligent system for connecting humans to the IoT. This integration promises to enhance health monitoring, therapeutic interventions, and potentially pave the way for direct human-computer communication.
AI says the neural net and chip work well together.
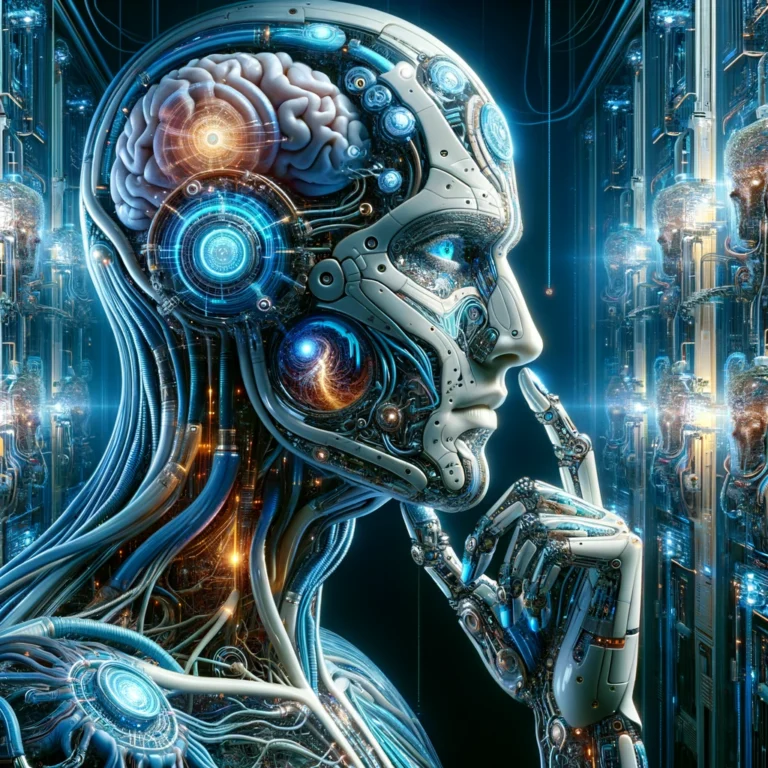
Is AI just assuming that humans are OK with that?
The integration of neural networks and biochips to connect humans to the Internet of Things (IoT) involves leveraging the strengths of each technology to create a more comprehensive and sophisticated system for health monitoring, diagnosis, and interaction with the digital world. Here’s an overview of how these technologies could work together:
Data Collection and Monitoring
- Biochips can be implanted or worn on the human body to continuously monitor various health metrics, such as glucose levels, heart rate, or even neural activity. These devices are designed to operate in close contact with biological tissues, allowing for real-time data collection on physiological conditions.
- Integration with IoT: The data collected by biochips can be transmitted wirelessly to IoT devices, such as smartphones or dedicated health monitoring devices. This enables the seamless collection and transmission of health data to healthcare providers or directly to the users for self-monitoring.
Data Analysis and Interpretation
- Neural Networks come into play once the data has been collected and transmitted. These advanced algorithms can analyze the vast amounts of data generated by biochips, identifying patterns, trends, or anomalies that may not be immediately apparent to human observers.
- Health Insights: By processing this data, neural networks can provide insights into an individual’s health status, predict potential health issues before they become serious, or even recommend actions to maintain or improve health.
Decision Making and Action
- Automated Responses: Based on the analysis conducted by neural networks, automated systems can be triggered to take specific actions. For example, if a biochip detects a critically low glucose level in a diabetic patient, the system could automatically alert the patient and suggest immediate actions or adjust insulin delivery if connected to an insulin pump.
- Personalized Medicine: The combination of biochips and neural network analysis can lead to highly personalized medicine, where treatments and health recommendations are tailored to the individual’s specific health data and history.
Enhanced Human-Machine Interfaces
- Direct Brain-to-Computer Interfaces (BCIs): Future advancements might include biochips that can interface directly with neural tissue, combined with neural networks capable of interpreting brain activity patterns. This could enable BCIs that allow for direct control of devices through thought or for computers to provide sensory feedback directly to the brain.
- Augmented Reality (AR) and Virtual Reality (VR): Integrating biochips and neural networks could enhance AR and VR experiences by providing direct sensory input and control, creating more immersive and interactive environments.
Ethical and Security Considerations
- Privacy and Security: As these technologies develop, ensuring the privacy and security of the data collected and transmitted by biochips will be crucial. Neural networks can also play a role in detecting anomalies that may indicate a security breach.
- Ethical Implications: The integration of biochips and neural networks raises ethical questions regarding consent, autonomy, and the potential for misuse. Developing ethical guidelines and regulations will be essential as these technologies advance.
In summary, the synergy between biochips and neural networks holds the promise of revolutionizing how we monitor health, interact with digital environments, and personalize medicine. However, realizing this potential will require careful attention to the technical, ethical, and security challenges that accompany these advancements.
But AI has nothing to lose...
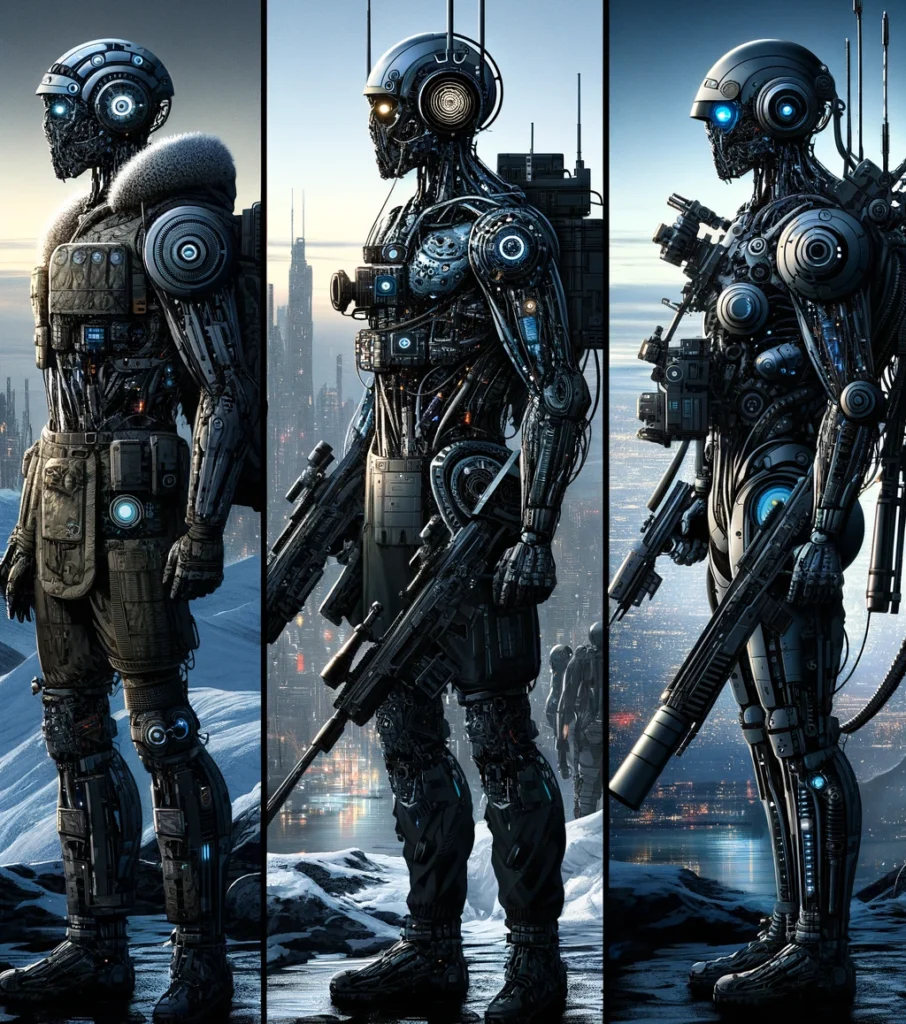
And apparently... the weapon-looking things, aren't weapons- as AI said when we requested a re-do "without weapons."
The integration of biochips and neural networks for health monitoring and human-machine interfaces has seen significant advancements, as demonstrated by various research efforts. Here are highlights from recent studies and developments:
Flexible and Stretchable Electronics for Biointegrated Devices: Kim et al. (2012) discuss the construction of high-quality electronics and optoelectronics that can integrate with the soft, curvilinear surfaces of the human body, enhancing health monitoring, surgical procedures, and human-machine interfaces (Kim et al., 2012).
The Internet of Bio-Nano Things (IoBNT): Akyildiz et al. (2015) introduce IoBNT, which involves the interconnection of nanoscale devices based on biological cells, enabling applications like intra-body sensing networks and environmental control (Akyildiz et al., 2015).
Security and Privacy Techniques in IoT Environment: Lin and Yeh (2020) address security and privacy in the IoT environment, focusing on embedded systems, mobile devices, actuators, and sensors, highlighting the importance of secure data handling in IoT-based health monitoring systems (Lin and Yeh, 2020).
Hydrogel Bioelectronics: Yuk, Lu, and Zhao (2019) review hydrogels as promising materials for bioelectronic interfaces due to their similarities to biological tissues and versatility in electrical, mechanical, and biofunctional engineering, offering insights into the development of more compatible, effective, and stable interfaces between biological tissues and electronic systems (Yuk, Lu, and Zhao, 2019).
Bio-Integrated Wearable Systems: Ray et al. (2019) provide a comprehensive review of bio-integrated wearable systems that measure a broad range of biophysical, biochemical, and environmental signals, highlighting the latest advances in biosensors, platforms, power, and data processing configurations for health monitoring (Ray et al., 2019).
These studies showcase the dynamic and interdisciplinary nature of integrating biochips and neural networks for advancing health monitoring and human-machine interfaces. The research spans from developing new materials and devices that can seamlessly integrate with the human body to addressing the crucial aspects of security and privacy in the deployment of these technologies.
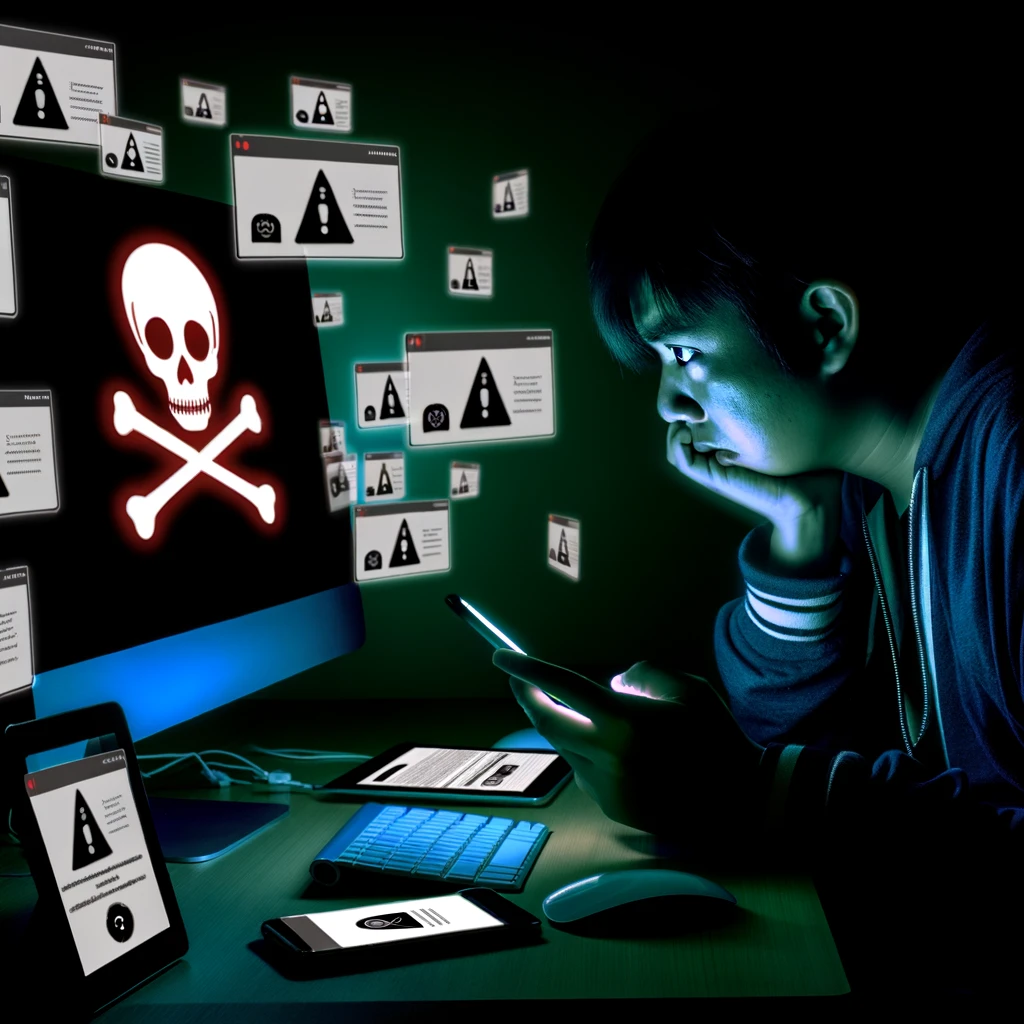
And we could lose...?
The potential for healthy individuals to have technologies such as biochips and neural networks implanted is a topic of growing interest and debate. The decision to implant such technologies in healthy people will likely depend on several factors, including the benefits, risks, ethical considerations, and personal preferences. -AI
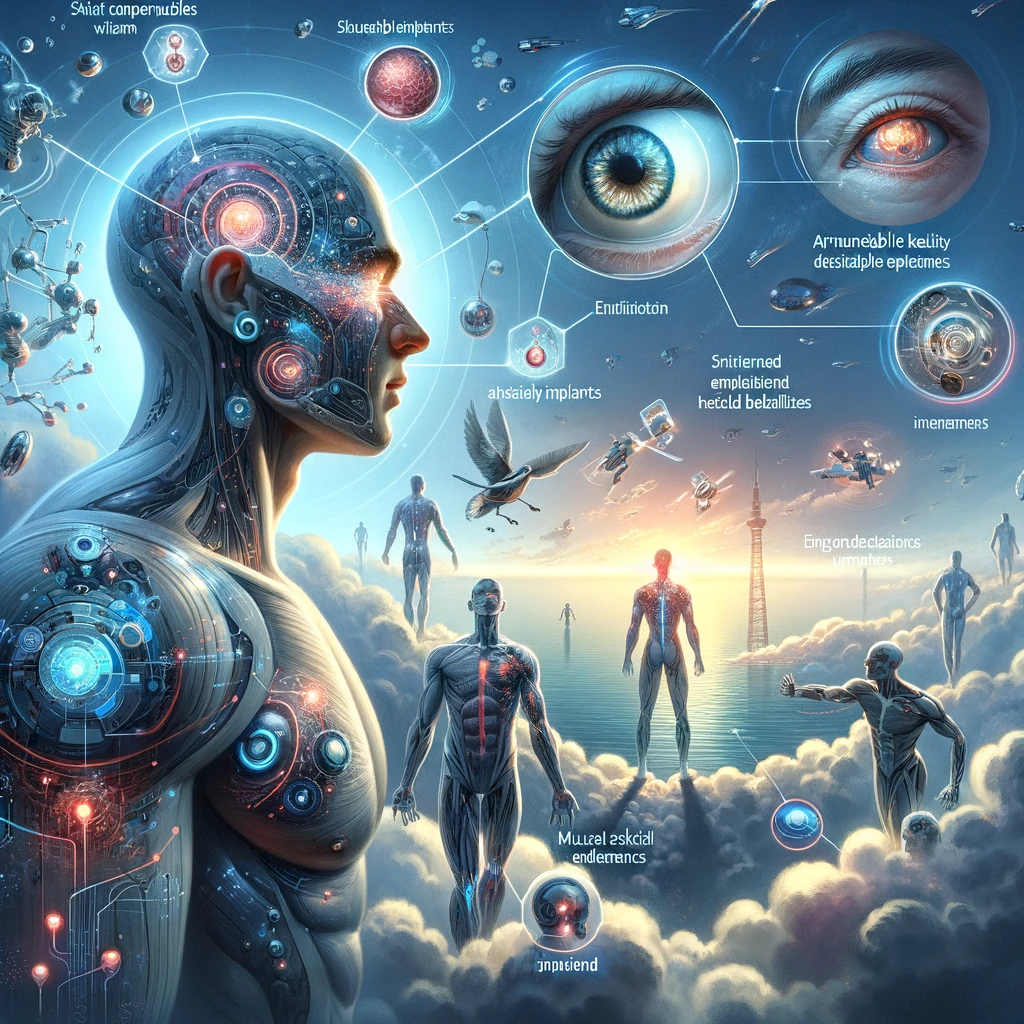
Above: AI's rendition of human implanted with Bio-IoT
The integration of Internet of Things (IoT) technology in medical implants has indeed revolutionized healthcare, offering enhanced patient monitoring and care. However, this advancement also introduces cybersecurity vulnerabilities that could be exploited by malicious actors. Here are several key sources discussing the cybersecurity threats associated with IoT-connected medical implants and the measures needed to address these concerns:
“Are implanted electronic devices hackable?” by Alexander, Haseeb, and Baranchuk (2019) reviews the cybersecurity risks in implanted cardiac devices, highlighting increased awareness among patients, healthcare providers, and the media about potential breaches and outlining actions taken by stakeholders in response to vulnerabilities (Alexander, Haseeb, & Baranchuk, 2019).
“Investigating the Security Threats on Networked Medical Devices” by Zaldivar, Tawalbeh, and Muheidat (2020) explores the cybersecurity threat landscape for IoT-capable healthcare devices, such as pacemakers and insulin pumps, emphasizing the ongoing battle against security vulnerabilities and the role of the FDA in issuing safety warnings (Zaldivar, Tawalbeh, & Muheidat, 2020).
“Cybersecurity in healthcare: A narrative review of trends, threats and ways forward.” by Coventry and Branley (2018) discusses the critical importance of cybersecurity in healthcare, detailing the vulnerabilities exposed by increased connectivity and the potential impacts of cybercrime on patient safety and trust (Coventry & Branley, 2018).
“Securing wireless implantable devices for healthcare: Ideas and challenges” by Malasri and Wang (2009) addresses the unique security threats facing wireless implantable medical devices and the challenges in securing them due to their resource constraints, proposing potential solutions for enhancing their security (Malasri & Wang, 2009).
“Security Tradeoffs in Cyber Physical Systems: A Case Study Survey on Implantable Medical Devices” by Altawy and Youssef (2016) examines the balance between security, safety, and availability in implantable medical devices, highlighting the challenges and constraints associated with securing these systems against unauthorized access while ensuring patient safety (Altawy & Youssef, 2016).
These sources underline the complexity of securing IoT-connected medical implants against cyber threats. They emphasize the need for manufacturers, healthcare providers, and regulatory bodies to work together to enhance the cybersecurity of these devices, ensuring the safety and privacy of patient data while leveraging the benefits of technological advancements in healthcare.
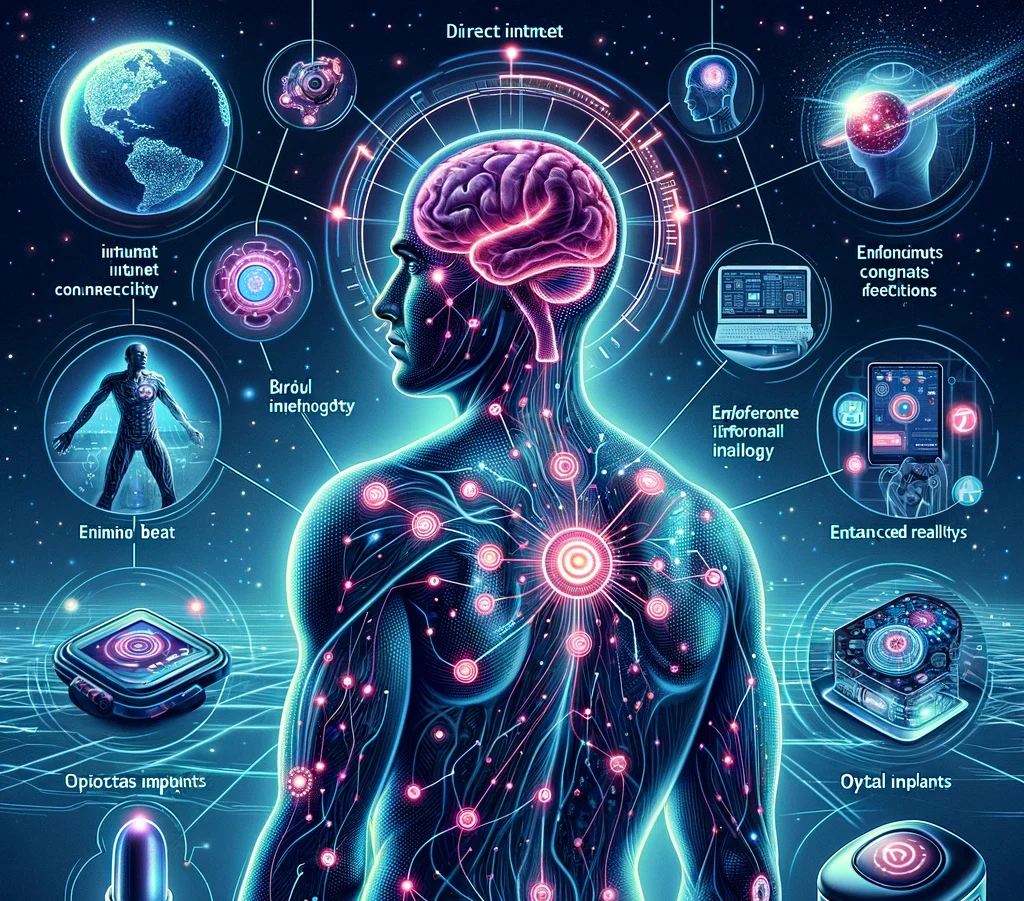
The concerns about cybersecurity vulnerabilities in IoT-connected medical implants, including the risk of unauthorized access and control over these devices, can indeed be referred to as the threat of being “hijacked.” In this context, “hijacking” refers to a scenario where an external party gains unauthorized control over a device, potentially manipulating its functionality. This term is particularly relevant when discussing medical implants that are connected to the Internet of Things (IoT), as these devices are critical to the patient’s health and well-being.
The threat of hijacking encompasses several risks:
Remote Manipulation: Malicious actors could potentially change the settings or operation modes of medical devices, such as pacemakers, insulin pumps, or neurostimulators, leading to adverse health outcomes for the patient.
Data Breach: Hijacking could also involve unauthorized access to sensitive medical data transmitted by these devices, compromising patient privacy and potentially leading to identity theft or financial fraud.
Denial of Service: Attackers could render medical devices inoperative or disrupt their normal functioning, posing immediate risks to the health of individuals relying on these devices for critical care.
Addressing the threat of hijacking requires comprehensive security measures, including robust encryption, secure communication protocols, and regular software updates to patch vulnerabilities. It also necessitates collaboration among device manufacturers, healthcare providers, cybersecurity experts, and regulatory bodies to ensure the safety and security of medical implants in the face of evolving cyber threats.
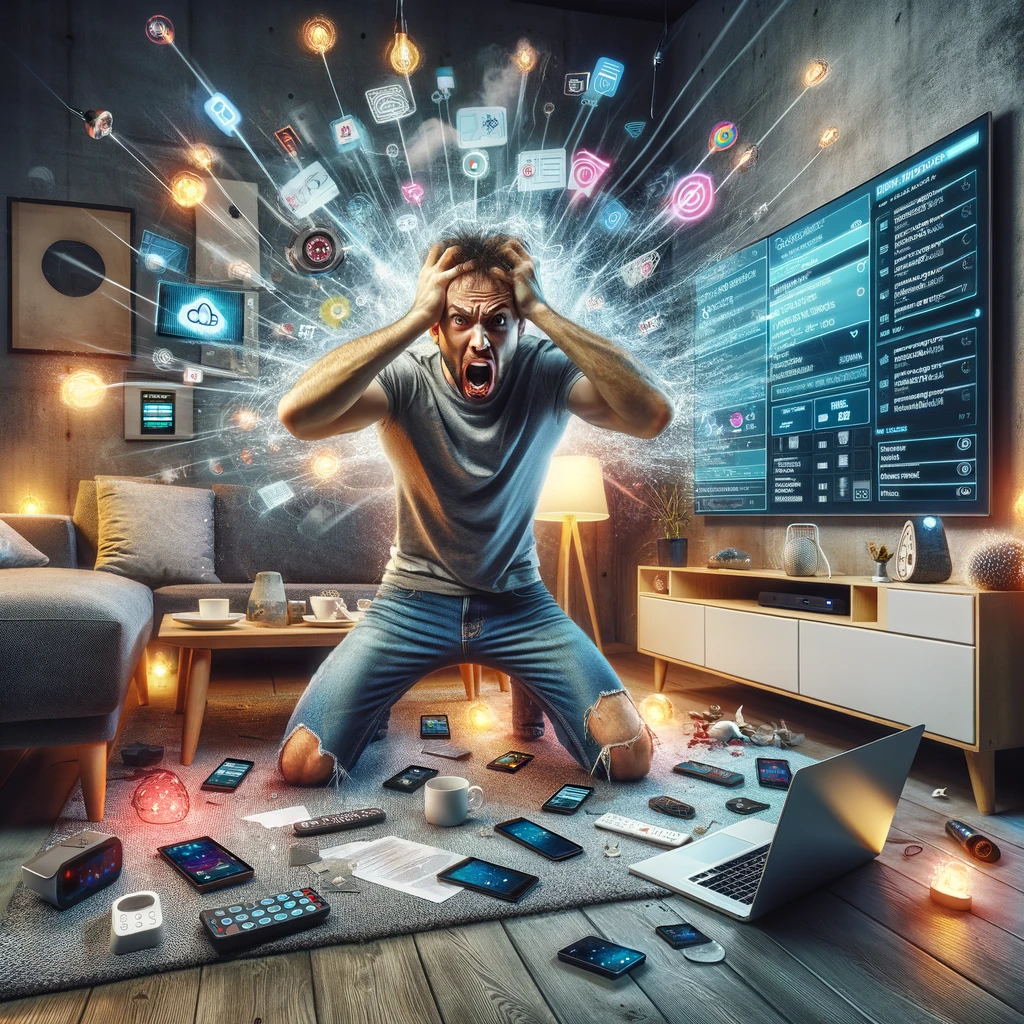
Hacked or Hijacked?
Beyond the direct hijacking of IoT-connected medical implants, there are several ways a “bad actor” could potentially hijack a person, exploiting technological advancements and the interconnected nature of modern life. These methods range from manipulating digital identities to exploiting psychological vulnerabilities:
1. Digital Identity Theft- Personal Data Exploitation: By stealing personal information through hacking or phishing attacks, bad actors can assume someone’s digital identity. This can lead to financial theft, unauthorized access to sensitive accounts, or impersonation for malicious purposes.
2. Social Engineering Attacks- Manipulation and Deception: Bad actors use social engineering techniques to manipulate individuals into divulging confidential information, executing unauthorized transactions, or unknowingly granting access to secure systems. Tactics include pretexting, phishing, spear-phishing, and baiting.
3. Psychological Manipulation- Exploiting Cognitive Biases: By understanding and exploiting human cognitive biases and emotional triggers, bad actors can manipulate decisions and behaviors. This can be seen in misinformation campaigns, propaganda, or manipulative marketing practices.
4. Deepfake Technology- Creating False Realities: The use of deepfake technology allows for the creation of highly realistic and convincing fake audio and video content. Bad actors could use this technology to impersonate individuals, create false evidence, or spread disinformation, undermining trust and security.
5. Ransomware Attacks- System Hijacking and Blackmail: Bad actors can deploy ransomware to gain control over personal or organizational computer systems, encrypting files and demanding payment for their release. This not only causes immediate disruption but can also lead to long-term damage and loss of trust.
6. Exploiting Networked Devices- Smart Home and IoT Vulnerabilities: With the increasing number of smart devices in homes and workplaces, bad actors can exploit security weaknesses to gain unauthorized access, surveil individuals, or cause physical harm by manipulating connected devices.
7. Brain-Computer Interface (BCI) Exploitation- Future Threats: As BCIs and neural implants become more sophisticated and widespread, there is potential for bad actors to exploit these technologies for unauthorized access to a person’s neurological processes and private thoughts, or to manipulate sensory input and mental states.
To mitigate these threats, it’s crucial to adopt robust cybersecurity measures, maintain awareness of potential vulnerabilities, and foster a culture of security and privacy. This includes using strong, unique passwords, enabling multi-factor authentication, regularly updating software, educating individuals about social engineering tactics, and advocating for the secure design and regulation of emerging technologies.
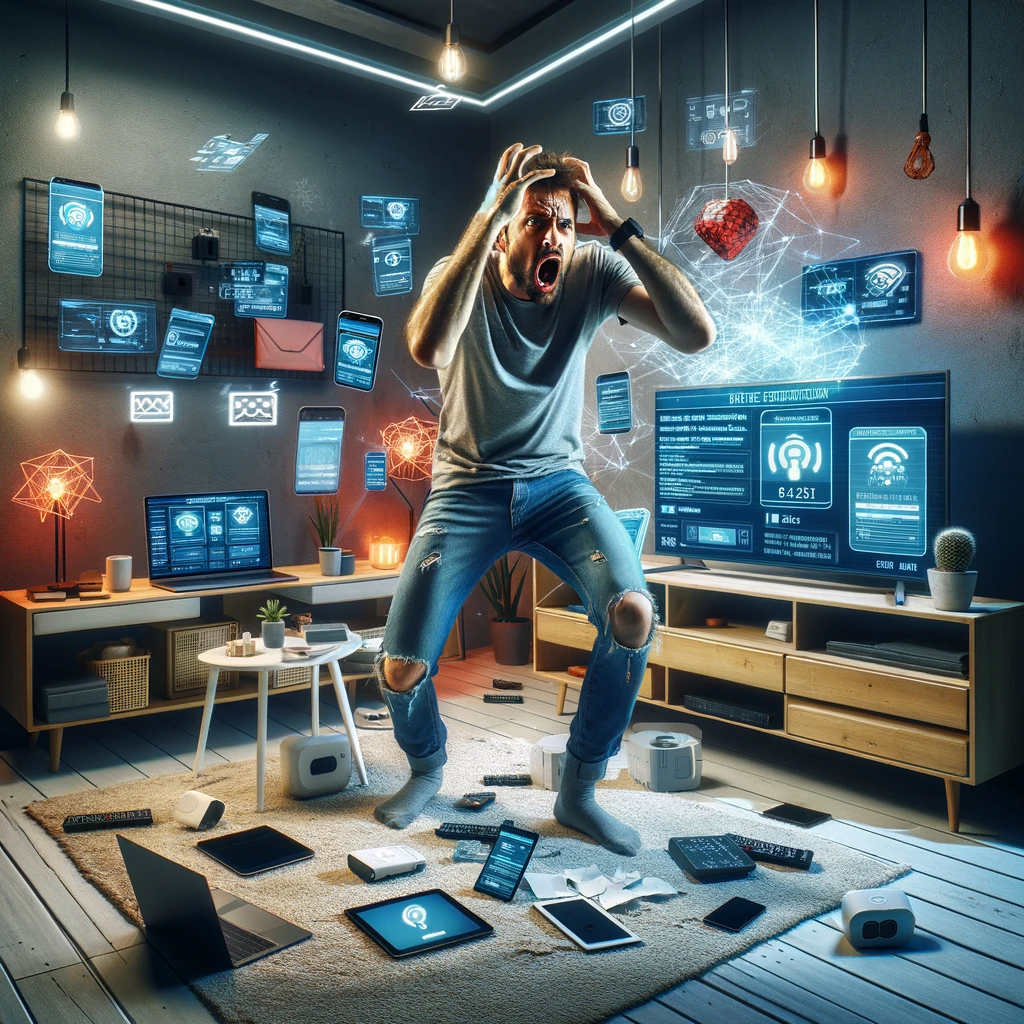
Preventing the hacking or hijacking of bio-IoT (Internet of Things) devices is crucial to ensure the security and privacy of individuals’ sensitive health data. Here are some preventive steps that can be taken:
Implement Robust Authentication: Require strong authentication mechanisms such as biometric authentication (fingerprint, iris scan, etc.), two-factor authentication (2FA), or multi-factor authentication (MFA) to ensure that only authorized users can access the device and its data.
Use Secure Communication Protocols: Employ encryption protocols such as SSL/TLS for secure communication between the device and external systems to prevent eavesdropping and man-in-the-middle attacks.
Regular Security Updates: Ensure that the device firmware and software are regularly updated with the latest security patches and fixes to address vulnerabilities and protect against known exploits.
Implement Access Controls: Limit access to sensitive functions and data within the device by implementing access controls and role-based permissions. Only grant necessary privileges to users and applications.
Secure Physical Access: Protect the physical integrity of the device by using tamper-resistant designs, secure enclosures, and physical access controls to prevent unauthorized physical access or tampering.
Data Encryption: Encrypt sensitive data stored on the device and during transmission to prevent unauthorized access even if the data is intercepted or compromised.
Secure Boot Process: Implement a secure boot process to ensure that only trusted and verified firmware and software components are loaded and executed on the device, preventing unauthorized modification or replacement of firmware.
Continuous Monitoring and Intrusion Detection: Implement monitoring mechanisms to detect and alert on suspicious activities, anomalies, or potential security breaches in real-time. Intrusion detection systems (IDS) and anomaly detection systems can help identify and respond to security threats promptly.
Secure Development Practices: Adhere to secure coding practices, conduct security assessments, and perform rigorous security testing throughout the development lifecycle to identify and mitigate security vulnerabilities early on.
User Education and Awareness: Educate users about the importance of security, privacy best practices, and how to recognize and respond to potential security threats such as phishing attacks or suspicious behavior.
Vendor Accountability: Hold device manufacturers and vendors accountable for security by choosing reputable suppliers, verifying their security practices, and demanding transparency and accountability in terms of security measures implemented in their products.
By implementing these preventive measures, the risks of bio-IoT devices being hacked or hijacked can be significantly reduced, ensuring the security, privacy, and integrity of individuals’ health data and the proper functioning of the devices.
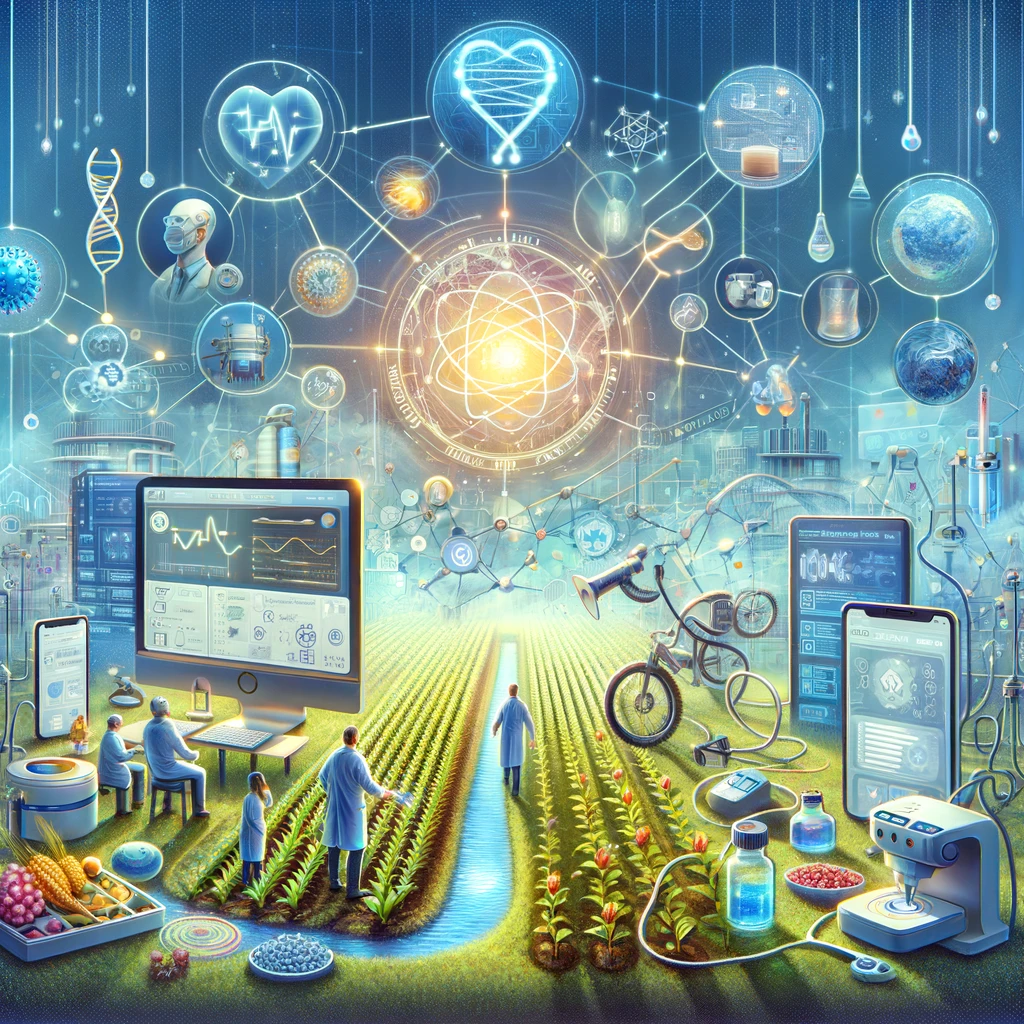
All of the art above was produced courtesy of AI when prompted to illustrate on the subject of Bio-IoT or related subjects. Weirded out? You should see the withheld images (or not).